by Paul Gilster | Feb 26, 2016 | Astrobiology and SETI |
When I first got interested in SETI, I naively assumed that we would get a detection fairly soon, and that we would detect not a directed beacon but simple background traffic in a remote civilization. I had no idea at the time how difficult it would be to pick up the kind of radio traffic we routinely generate on Earth from a distant star, and as a matter of fact, my interest in shortwave radio led me to assume that, just as I enjoyed the sport of DX — listening for distant signals — so SETI would simply be an offshoot of this, with a harder-to-get QSL card.
Some time in the mid-1980s I wrote a piece called “Where the Real DX Is” for Glenn Hauser’s Review of International Broadcasting, running through a list of the nearest stars and talking about SETI projects that had been tried up to then. I haven’t gone back to read that article in years and would probably find it an embarrassing chore. But it’s interesting to me that the idea of leakage radiation does have its place, particularly in the area of beamed power.
Power Beaming at Work
What I knew little of back in the 80’s was how beamed power — using high-power microwave beams but including millimeter-wave beams and lasers — might empower a space-based infrastructure. While television and radio signals are all but undetectable from another star (at least, for a civilization at our level of development), intense, focused beams to impart power to a spacecraft present a better target. The interesting question that James Benford (Microwave Sciences) and son Dominic (NASA GSFC) ask in the paper we began looking at yesterday is just how detectable power beaming would be.
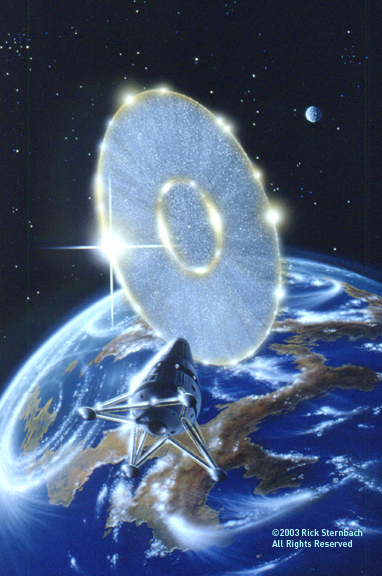
This takes off on ideas Jim Benford discussed last year in these pages (see Seeing Alien Power Beaming), and both Jim and Dominic recently looked at the question in relation to SETI efforts on a very intriguing star indeed in Quantifying KIC 8462852 Power Beaming. After all, if a distant civilization were building something like a Dyson sphere or ‘swarm’ around a star, it would have to have constructed an infrastructure that might well involve power beaming technologies.
Image: A favorite image from a favorite artist, Rick Sternbach. Here we’re looking at an interstellar lightsail near an exotic world. The uses of beamed power in near and deep space are numerous, and many of them appear to be detectable from a SETI perspective. The image comes from a 1983 issue of Science Digest. Credit: Copyright Rick Sternbach.
The Benford paper runs through a number of beaming concepts ranging from orbit raising, a lower power application, to actual launch to orbit using microwave thermal thrusters. Various high-power beaming applications have been quantified for missions within the Solar System as well, using microwave or laser beams to boost craft to 100 to 200 kilometers per second. A mature infrastructure might involve high-speed unmanned supply craft being decelerated upon arrival by a beam system similar to the one that launched it. I mentioned yesterday that beaming from a solar power station in space to a planetary surface is not a likely observable. Here’s why, whether we’re talking about microwave bands or lasers in the optical range:
The beam must be carefully controlled to deliver power to the receiving rectifying antennas on the ground (Mankins 2014). Any side emissions are economic losses, therefore substantial measures would be taken to reduce side lobes to a minimum. Further, the first several sidelobes are absorbed in the ground. The remaining side lobes are dispersed in angle so that the power density in the far field will be very low. For the worked example in Mankins (Mankins 2014; Dickinson 2016), the back lobe is down 40 dB relative to the ? 1GW main beam.
And note this:
This is in contrast to power beaming transportation applications, in which the varying solid angle of the receiving spacecraft results in the main beam increasingly leaking around the edges of the vehicle being accelerated.
So that takes detection of one of the numerous power beaming scenarios off the table, but many others survive scrutiny. Have a look at this table from the paper, which draws on representative parameters for applications of power beaming presented earlier in the discussion.
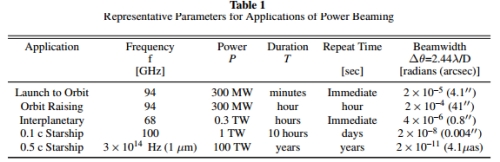
Here we’re looking at observables in terms of slew rate (movement of the beam), the EIRP and the observation time. EIRP stands for Effective Isotropic Radiated Power, the measured radiated power in a single direction — it’s the product of the antenna gain times the power radiated. For useful background, the standard text is Jim Benford’s own High Power Microwaves (now in its third edition, CRC Press, 2016). A quick note on EIRP from the paper:
Spectral flux density, typically denoted in Janskys, is the power density divided by the bandwidth. While this is commonly used as the observed quantity in radio astronomy, we cannot know the bandwidth of an ETI transmitter. Consequently, in thinking about ETI power beaming mission [sic] we must deal with EIRP, not spectral flux density. Beaming power does not require or even necessarily benefit from narrow bandwidth; energy transference is what matters.
Ranking the Observables
The possibilities are numerous, and not all of them designed for deep space. Microwave thermal thrusters, for example, can be fed by a high-power microwave beam in a single-stage rocket with a flat aeroshell on the underside covered by microwave-absorbing heat exchangers. The system is efficient because the energy source remains on the ground — see the paper for further parameters on this as well as orbit raising, where ground-based energy sources lift a satellite to a higher orbit. As the table shows, orbit raising is significantly lower in power than launch from the surface.
But of course beaming applications deeper into the system are a part of a robust infrastructure based on beaming, and here we can look at interplanetary transfers by beam-driven sails, as well as starship concepts involving prolonged acceleration of an interstellar vehicle, like the Robert Forward concepts I mentioned yesterday. Given the power requirements for starships, such beams would be detectable with our current technology, but this would also require that the Earth fall within the bandwidth of the power beam.
These questions of detectability depend, of course, upon the level of technology of the civilization trying to make the detection. In our case, we have the interesting story of the star KIC 8462852 to give us some guidance. We have radio observations of this curious star that found no signs of power beaming in about 180 hours of observations. The idea was to look for incidental radiated power — leakage radiation — produced by an advanced civilization. The observations were made using the Allen Telescope Array in the 1-10 GHz range.
Refer back to the table above and the beaming applications there. These are the authors’ conclusions re the KIC 8462852 observations using the Allen Telescope Array:
- The 1 Hz channels could see all the applications, but they are not seen.
- Launch from a planetary surface into orbits is marginally detectable, at the threshold of the Allen Array for the 100 kHz observations, if at the frequencies observed. Orbit raising, which requires lower power, is not detectable.
- Interplanetary transfers by beam-driven sails should be detectable in their observations, but are not seen. This is for both the 1 Hz and for the 100 kHz observations.
- Starships launched by power beams with beamwidths that we happen to fall within (to other solar systems, not our own) would be detectable, but are not seen.
We also learn that the limited observation times and wavelength coverage mean that we have no firm conclusions about the star. More systematic studies, which would include observations at higher radio frequencies, would be called for to come up with a stronger result. I should also mention that an optical SETI attempt was made on KIC 8462852 at the Boquete Optical SETI Observatory in Panama. Given the detection limits of the equipment there and other issues discussed in the paper, none of the power beaming applications in consideration by the Benfords could have been detected at the Boquete site.
The authors’ conclusion:
*As discussed above, the beaming power levels are high and transient and easily dwarf any ETI civilization’s diffuse leakage to space (Sullivan 1978). Power beaming described here is larger than that necessary for beaming systems for communication: EIRP = 1018 W for a 1,000ly-range beacon (Benford, Benford & Benford 2010). SETI programs could explore a different part of parameter space by observations suitable to finding leakage from power beams.
We have no way of knowing whether any civilization exists outside of our own, nor can we know if such a civilization would use power beaming. But the virtues of beamed power for fast transportation within our own Solar System — and for lowering the cost to orbit, as well as raising orbits — give us good reason to continue to explore the use of this technology. In doing so, we should keep in mind that we are producing an observable signal that is well beyond those undetectable “I Love Lucy” episodes that are now 65 light years out. I think the Benfords are right when they argue that detectable radiation is a message in itself, whether or not we ever decide to impose a structured message into the beams that power a future infrastructure.
The paper is Benford & Benford, “Power Beaming Leakage Radiation as a SETI Observable,” submitted to The Astrophysical Journal and available as a preprint.
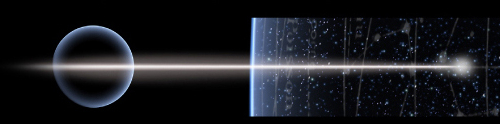
by Paul Gilster | Feb 25, 2016 | Astrobiology and SETI |
Thinking that we can understand the motivations of an extraterrestrial civilization seems like a fool’s gambit, but we have to try. The reason is obvious: We have exactly one technological society to work with — we’re all we have — and if we want to look for SETI signals, we have to interpolate as best we can. An alien culture, it is assumed, will do the same. This was the procedure outlined by Giuseppe Cocconi and Philip Morrison in their classic 1959 paper “Searching for Interstellar Communications,” that began the modern era of SETI.
If there are civilizations around stars like the Sun, the paper reasons, then some will be motivated to reach out elsewhere. From the paper:
To the beings of such a society, our Sun must appear as a likely site for the evolution of a new society. It is highly probable that for a long time they will have been expecting the development of science near the Sun. We shall assume that long ago they established a channel of communication that would one day become known to us, and that they look forward patiently to the answering signals from the Sun which would make known to them that a now society has entered the community of intelligence. What sort of a channel would it be?
What results is an explanation of the factors needed to overcome signal attenuation and the frequencies most likely to be used. Thus we get what Cocconi and Morrison called “a unique, objective standard of frequency, which must be known to every observer in the universe.” This is 1420 MHz, the 21 centimeter wavelength of neutral hydrogen. Thus we know where to look assuming a civilization is trying to make contact with us. The authors conclude “… the foregoing line of argument demonstrates that the presence of interstellar signals is entirely consistent with all we now know, and that if signals are present the means of detecting them is now at hand.”
The Hunt for SETI Observables
We’ve looked frequently in these pages at how SETI has changed since the Cocconi and Morrison days, with so-called ‘Dysonian SETI’ invoked as a way of looking for observable evidence in our astronomical data. The unusual star KIC 8462852 has elevated the method to wider attention because of the possibility that some kind of astroengineering could explain its unusual light curves (search the archives here for numerous articles on the star).
In any case, Dysonian SETI assumes, contra Morrison and Cocconi, no intent to contact anyone. We are simply looking for activity, albeit on a colossal scale. We must be the ones to figure out what that activity might be.
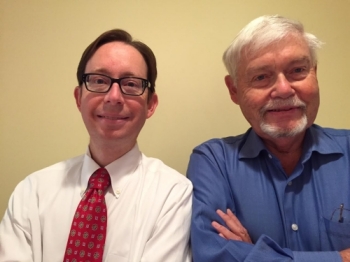
Which brings me to a new paper from James Benford and son Dominic (NASA GSFC). “Power Beaming Leakage as a SETI Observable,” submitted to The Astrophysical Journal, asks whether we might detect the use of power beaming to transfer energy and accelerate spacecraft within a distant solar system and, perhaps, beyond it if the technology is being used to accelerate starships. KIC 8462852 is also considered here because there have been two attempts to study it, one optical and one at radio frequencies, in the context of a SETI search.
As the paper notes:
The most observable leakage from an advanced civilization may well be from the use of power beaming to transfer energy and accelerate spacecraft, both within and beyond the star system where the civilization is located. In future, such applications may make the Earth’s radiation in the microwave, millimeter and visible/near-IR parts of the electromagnetic spectrum be very intense…. The power levels are high, focused, and transient and could easily dwarf any of our previous leakage to space. These are not SETI signals so much as leakage, a detectable aspect of advanced civilizations.
The kind of SETI observable the Benfords are examining is essentially leakage, but of a different kind than the widely repeated trope of picking up alien TV signals, just as extraterrestrial cultures are presumably now enjoying “I Love Lucy” from our signals. As the Benfords argue, the leakage of radio and television signals is essentially undetectable between stars not only due to the weakness of the signal but to its lack of coherence. Planetary radars are much more likely to be detectable, but signals like these are also extremely transient.
Intense beams of radiation being used to move power about in a distant solar system or accelerate spacecraft on long journeys should be more readily detected. James Benford argued as much in 2008, and more recently James Guillochon and Abraham Loeb have quantified the leakage to be expected from space propulsion-related beaming, showing that a beam being used to drive a large sail would be observable. The duo write: “…for a five-year survey with ~10 conjunctions per system, about 10 multiply-transiting, inhabited systems would need to be tracked to guarantee a detection” using our existing radio telescope infrastructure.
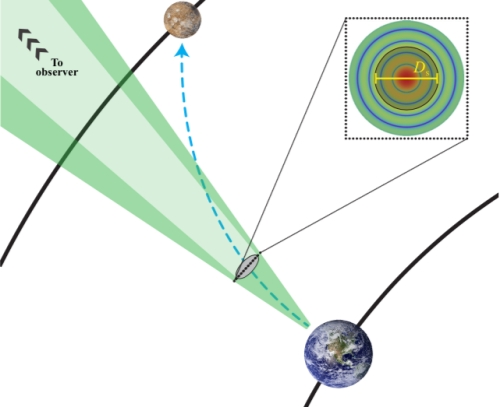
Image: Taken from the Guillochon and Loeb paper, this diagram shows a Mars mission using microwave beaming for propulsion. In the schematic, the dashed line represents the sailcraft. You can see the prospects for signal leakage here. Notice the inset showing the beam profile as it overlaps the sail of diameter Ds. Credit: Guillochon & Loeb.
But remember the notion we started with: If we are trying to understand what an extraterrestrial civilization might do, we have to look at the uses we ourselves would make of these technologies. We try to be as flexible as possible while acknowledging the fundamental gap in our knowledge of an alien culture, but we have to start somewhere. And this kind of reasoning takes us down an interesting path, as the Benfords note in their paper. For possessed of powerful beaming technologies, such a culture should be aware of SETI implications:
It has previously been noted that such leakage from other civilizations could be observable (Benford 2008). Guillochon & Loeb (2015) have quantified leakage from beaming for interplanetary space propulsion, its observables, and implications for SETI. Extraterrestrial Intelligence (ETI), having done the same thinking, could realize that they could be observed. Hence there may be a message on the power beam, delivered by modulating it in frequency, amplitude, polarization, phase, etc., and broadcast it for our receipt at little additional energy or cost. By observing leakage from power beams we may well find a message embedded on the beam.
That’s a fascinating notion in its own right. It’s a kind of METI signal sent out by an alien civilization that is, like a beacon, broadly targeted rather than aimed at a specific solar system. And it operates on the assumption that another culture might in the course of its SETI investigations notice high-powered, focused and transient beams and analyze them. Or put another way, if our own civilization has figured this out, our extraterrestrial counterparts working the SETI various concepts must surely have come up with the same implications.
Uses of the Beam
Detecting a message embedded in a power beam would, of course, be a breakthrough of historic proportions, but so would the simple detection of power-beaming itself — no message attached — which would signal the presence of an advanced civilization. One of the things recently looked at by the Allen Telescope Array was whether the intriguing star KIC 8462852 showed any signs of activity, and it turns out that the observations, while finding nothing, do allow us to set some limits on power-beaming in that system. KIC 8462852 became, in other words, a useful exercise even though the observations were short-lived. More tomorrow on this, and also see the Benfords’ Quantifying KIC 8462852 Power Beaming in these pages.
And just what might a technological civilization do with power-beaming methods that we could observe? One answer is obvious: We’ve been talking about beamed sails for well over ten years on Centauri Dreams, keying off Robert Forward’s fascination with the subject as it applied to interstellar missions. Here the requirements are enormous, with a space-based solar power station beaming to a 1000-kilometer sail in some of Forward’s missions, although subsequent work found ways to trim the sail down to the 1-10 kilometer range.
The idea of a power station orbiting in the inner part of the Solar System where the photon-flow is maximized has dual use, as using microwave beams to transport power to a planet’s surface is a major driver. As a SETI observable, power beaming to a planet is not a likely target. The paper notes that the beam would have to be tightly controlled, with side lobes maximally reduced. In contrast, power being beamed to a spacecraft should show increasing leakage as the craft is accelerated — the beam increasingly leaks around the edges of the accelerating vehicle — making transportation applications a realistic SETI observable.
The Benfords’ paper quantifies the various kinds of power beaming missions and applications and their observable parameters, looking at twenty concepts in terms of power radiated, duration and likely time for the radiation to repeat. More on this tomorrow as we continue looking at the paper and the question of how an advanced technology could use power beaming.
The Benfords paper is “Power Beaming Leakage Radiation as a SETI Observable,” submitted to The Astrophysical Journal and available as a preprint. The Guillochon and Loeb paper is “SETI via Leakage From Light Sails in Exoplanetary Systems,” The Astrophysical Journal 811, No. 2 (23 September 2015), with abstract here. Jim Benford also discusses the Guillochon and Loeb paper in Seeing Alien Power Beaming.
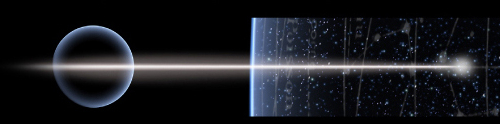
by Paul Gilster | Feb 24, 2016 | Deep Sky Astronomy & Telescopes |
Have we finally traced a Fast Radio Burst to its place of origin? News from the CSIRO (Commonwealth Scientific and Industrial Research Organisation) radio telescopes in eastern Australia, along with confirming data from the Japanese Subaru instrument in Hawaii, suggests the answer is yes. Fast Radio Bursts (FRBs) are transient radio pulses that last scant milliseconds. In that amount of time, they have been known to emit as much energy as the Sun emits in 10,000 years. And exactly what causes FRBs is still a mystery.
Take the so-called ‘Lorimer Burst’ ( FRB 010724) which was discovered in archival data from 2001 at the Parkes radio telescope in New South Wales. Here we’re dealing with a 30-jansky dispersed burst that was less than 5 milliseconds in duration. Although the burst appeared roughly in the direction of the Small Magellanic Cloud, the FRB is not thought to be associated with our galaxy at all. A 2015 event, FRB 110523, was discovered in data from the Green Bank dish in West Virginia, with an origin thought to be as much as six billion light years away.
Sixteen FRBs have been detected, but according to this news release from the Max Planck Institute for Radio Astronomy, researchers believe they are a much more common phenomenon. A new paper in Nature now tells us that the Parkes telescope detected a new Fast Radio Burst (FRB 150418)) on April 18, 2015. But in this case, we have a twist. Evan Keane (Square Kilometre Array Organisation, Jodrell Bank UK) and an international team have been developing a system to detect FRBs within seconds, which makes it possible to alert other telescopes to pinpoint the source.
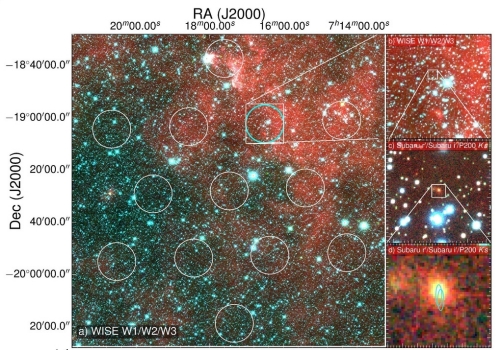
Image: The infrared image on the left shows the field of view of the Parkes radio telescope with the area where the signal came from marked in cyan. On the right are successive zoom-ins on that area. At the bottom right is the Subaru optical image of the FRB galaxy, with the superimposed elliptical regions showing the location of the fading 6-day afterglow seen with ATCA. Credit: © D. Kaplan (UWM), E. F. Keane (SKAO).
The system, part of the Survey for Pulsars and Extragalactic Radio Bursts (SUPERB), allows quick detection and transmission of the relevant information to other telescopes. Using it, within two hours of the Parkes detection the Australia Telescope Compact Array (ATCA) 400 kilometers to the north was able to trace the FRB to a radio source that lasted six days before finally fading out. What the Subaru telescope adds to the mix was that it found a galaxy at optical wavelengths that matches up with the radio source detected by the Compact Array. Unlike the previous sixteen detected bursts, we now have a far more precise fix on a location.
The culprit turns out to be an elliptical galaxy with a redshift consistent with a distance of six billion light years. This marks the first time a host galaxy has been determined for an FRB, and the first time an FRB’s distance has been measured. Simon Johnston (Head of Astrophysics at CSIRO) notes that the galaxy in question is well past its prime period for star formation, an unexpected result. Johnston speculates that the cause of the FRB may be two neutron stars colliding rather than any process associated with the birth of young stars.
Whatever causes them, Fast Radio Bursts turn out to be useful in interesting ways. They show a frequency-dependent dispersion, which means the radio signal is affected by the amount of material it has moved through. An FRB thus makes it possible to conduct a cosmological measurement. Says Johnston:
“Until now, the dispersion measure is all we had. By also having a distance we can now measure how dense the material is between the point of origin and Earth, and compare that with the current model of the distribution of matter in the universe. Essentially this lets us weigh the universe, or at least the normal matter it contains.”
The result: A universe made up of 70 percent dark energy, 25 percent dark matter and 5 percent ordinary matter is a good fit for the measurement conducted on the FRB pulse. What’s intriguing here is the prospect that we are moving into an era of FRB astronomy. As we detect more and more of these events, we may find new ways to investigate cosmological questions.
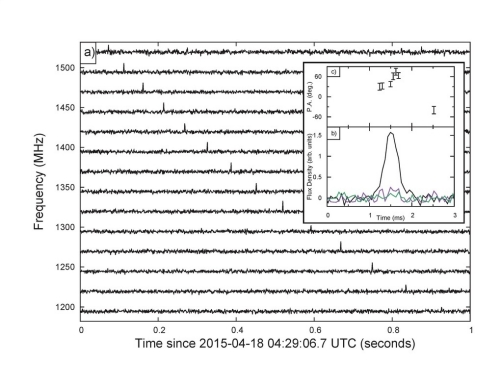
Image: This image shows the increased delay in the arrival time of the Fast Radio Burst as a function of the frequency. The delay in the signal is caused by the material it goes through between its point of origin in a distance of 6 billion light years and Earth. Credit: © E. F. Keane (SKAO).
To be sure, we still don’t know what causes FRBs. But as Johnston points out, the Australian SKA Pathfinder (ASKAP) is going to be able to start looking for FRBs later this year. ASKAP is a next-generation radio telescope made up of 36 identical 12-meter antennas that will work together as an interferometer. The fully implemented Square Kilometre Array is likewise expected to detect numerous FRBs and pinpoint their locations. We should soon have abundant data to pursue questions of FRB origin and the cosmological issues the bursts can illuminate.
The paper is Keane et al., “The Host Galaxy of a Fast Radio Burst,” Nature 530 (25 February 2016), 453-456 (abstract).
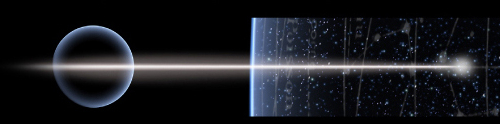
by Paul Gilster | Feb 23, 2016 | Exoplanetary Science |
Keep your eye on a program called the Hubble Cloud Atlas. This is a collaboration between fourteen exoplanet researchers around the globe that is intent on creating images of exoplanets using the Hubble Space Telescope. But while we’ve been able to directly image a small number of planets before now, the Cloud Atlas project brings a new twist. The plan is to create time-resolved images that can tease out details about planetary atmospheres.
The test case is the planet 2M1207b, about 160 light years out in the constellation Centaurus. Infrared imaging made it possible to directly observe this planet in April of 2004, a task accomplished by researchers from the European Southern Observatory using data from the Very Large Telescope at Paranal (Chile). What we know about this planet makes it a formidable — and definitely uninhabitable — object, one with a surface temperature in the 1700 K range.
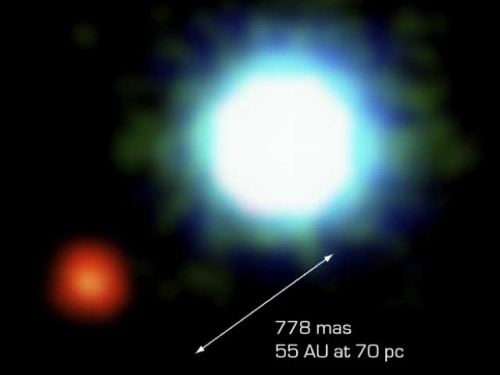
Image: The 2M1207 star system, showing the faint red object 2M1207b, a planet four times the mass of Jupiter whose atmospheric properties are now under investigation. This is an earlier image — not part of the Hubble work — taken with the high-resolution adaptive-optic NaCo camera attached to the 8-meter Very Large Telescope Yepun instrument in Chile. Credit: ESO.
At four times the mass of Jupiter, 2M1207b orbits a brown dwarf at a distance roughly comparable to Pluto’s from the Sun. Its searing heat, then, isn’t due to proximity to the brown dwarf but to gravitational contraction. This is a planet perhaps only ten million years old, and as it continues to contract, its rain likely falls in the form of liquid iron and glass. University of Arizona graduate student Yifan Zhou, lead author of the paper on this work, has been studying the distant world by taking 160 Hubble images over the course of a ten hour run.
Hubble’s Wide Field Camera 3, with high-resolution and high-contrast imaging capabilities, makes the work possible, but this is the first time anyone has used the space telescope to create time-resolved images of an exoplanet. This becomes a way of mapping a planet’s clouds even without being able to see them in sharp relief. Instead, the researchers measure the changes in brightness that occur over time as the planet completes its 11-hour rotation.
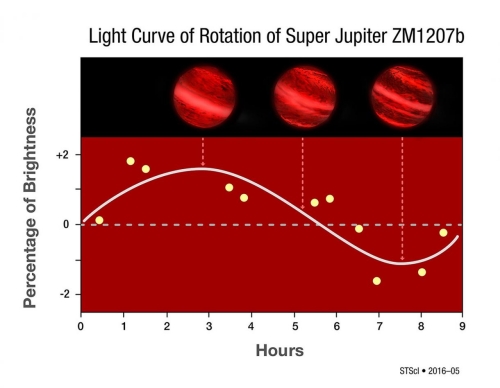
Image: This graph shows changes in the infrared brightness of 2M1207b as measured by the Hubble Space Telescope. Over the course of the 10-hour observation, the planet showed a change in brightness, suggesting the presence of patchy clouds that influence the amount of infrared radiation observed as the planet rotates. Credit: NASA, ESA, Y. Zhou (University of Arizona), and P. Jeffries (STScI).
Data collection for the Hubble Cloud Atlas program began in 2014. Daniel Apai (University of Arizona), who is lead investigator for the program, describes it this way on his website:
The program is using high-precision, time-resolved photometry and spectroscopy of these rotating objects to derive course maps of their cloud structures. By comparing the cloud maps between different objects we can determine how the cloud properties (covering fraction, cloud thickness, vertical structure) depend on the upper atmosphere’s temperature and the surface gravity of the objects.
And from the paper:
… we note that the observations presented here open an exciting new window on directly imaged exoplanets and planetary-mass companions. Our study demonstrates a successful application of high contrast, high-cadence, high-precision photometry with planetary mass companion. We also show that these observations can be carried out simultaneously at multiple wavelengths, allowing us to probe multiple pressure levels. With observation of a larger sample and at multiple wavelengths, we will be able to explore the detailed structures of atmospheres of directly imaged exoplanets, and identify the key parameters that determine these.
Zhou’s work with 2M1207b, which began in 2014, has bolstered the new Hubble Cloud Atlas program, and points to how we can further use Hubble as well as the upcoming James Webb Space Telescope to study planetary atmospheres and map cloud structures. Zhou was able to deduce the planet’s rotational period and probe its atmospheric properties in ways that existing Earth-based telescopes cannot. As our catalog of directly imaged planets grows, we’ll acquire new targets with which to hone these methods and eventually apply them to smaller worlds.
The paper is Zhou et al., “Discovery of Rotational Modulations in the Planetary-Mass Companion 2M1207b: Intermediate Rotation Period and Heterogeneous Clouds in a Low Gravity Atmosphere,” The Astrophysical Journal Vol. 818, No. 2 (18 February 2016). Abstract / preprint available.
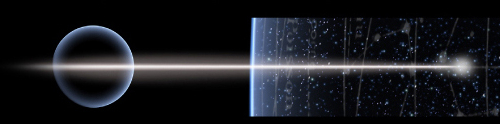
by Paul Gilster | Feb 22, 2016 | Astrobiology and SETI |
Given the interest the unusual star KIC 8462852 has generated here and elsewhere, I want to be sure those of you in California are aware of an upcoming talk that touches on the matter, as well as broader SETI issues. Titled “The Breakthrough Initiative – Listen and Megastructures at KIC 8463,” the talk will be delivered by Andrew Siemion (UC-Berkeley). The venue is 1065 La Avenida Street, Mountain View, CA 94043. The time: Tuesday, February 23, 2016 from 12:00 PM to 1:00 PM (PST).
More at this web page, from which the description that follows:
Dr. Andrew Siemion, Director of the Berkeley SETI Research Center (BSRC) at the University of California, Berkeley, will present an overview of the Breakthrough Listen Initiative, 100-million-dollar, 10-year search for extraterrestrial intelligence. Dr. Siemion will also discuss other SETI efforts ongoing at the BSRC, including the successful citizen science project SETI@Home, as well as a concerted effort to undertake panchromatic observations of the mysterious Kepler star KIC 8462852.
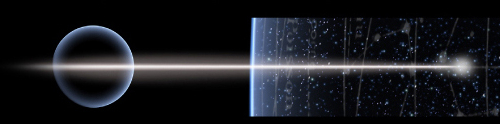