by Paul Gilster | Jan 30, 2017 | Astrobiology and SETI |
Is it possible to use natural phenomena to boost signals to the stars? In the essay below, Bill St. Arnaud takes a look at the possibilities, noting that civilizations that chose to broadcast information might select a method that mimics by electromagnetic means what the classic von Neumann probe would achieve with physical probes. St. Arnaud is an optical communications engineer, a network and green IT consultant who works with clients on a variety of subjects such as next generation research/education and Internet networks. His interest in practical solutions — free broadband and dynamic charging of electric vehicles — to reduce greenhouse gas emissions is matched by a fascination with interstellar matters, particularly SETI.
By Bill St. Arnaud
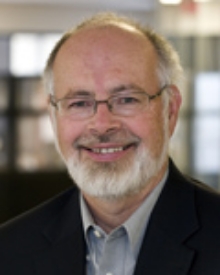
In their recent post on Centauri Dreams Roger Guay and Scott Guerin (https://centauri-dreams.org/?p=36802) make a compelling argument that fading electromagnetic halos may be all that’s left for us to discover of an extraterrestrial civilization. They argue that there is only a short window in the evolution of a sufficiently intelligent species in which it will broadcast its presence through inefficient electromagnetic transmission of radio, TV and radar signals.
Current SETI searches assume that an advanced civilization will use extremely powerful omnidirectional transmitters or highly directional and focused beacons targeted at our solar system. The challenge with either approach is the fact that successful one-way communication between intelligent species is dependent on the “L” term in the Drake equation. L represents the length of time for which such civilizations release detectable signals into space. If L is relatively short then the possibility of two separate intelligent civilizations being coincident in time to send and receive a signal is very small, as demonstrated by Guay-Guerin. So even though there may have been many intelligent civilizations we will probably never be aware of their existence.
On the other hand, Stephen Webb, in his book If the Universe is Teeming with Aliens .. Where Is Everybody? argues that we may be the only intelligent civilization in our galaxy, if not perhaps the known universe. This is often referred to as the Rare Earth Hypothesis. Given the large multiplier of improbabilities from the creation of simple life, through the prokaryotic-eukaryotic transition, and the many divergent evolutionary pathways in human evolution leading to technology savvy beings, Webb argues that the odds of this being replicated elsewhere in the universe are extremely low.
The bottom line from both Guay-Guerin and Stephen Webb is that regardless of whether the universe is teeming with advanced civilizations or limited to only a very few it would seem that the probability of detecting a SETI signal by conventional means is very limited.
In another line of reasoning in SETI exploration it has been suggested that we look for physical artifacts as well as electromagnetic signals. These artifacts could include devices like Von Neumann probes and physical remains of past advanced civilizations.
A Von Neumann probe is a self replicating spacecraft that would travel from stellar system to stellar system through a galaxy where it would seek out raw materials extracted from planets to create replicas of itself. These replicas would then be sent out to other stellar systems.
Searching for small physical artifacts such as Von Neumann probes would seem to be even more daunting than looking for the proverbial “needle in the haystack” electromagnetic signals. If a civilization were advanced enough to launch artifacts through space you would think at some earlier stage in its existence it would have initially deployed electromagnetic beacons or omnidirectional broadcasts. Of course this is assuming that they do want to make their presence aware to other advanced civilizations.
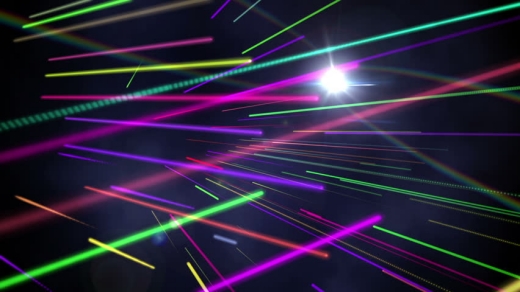
Von Neumann Signaling
But perhaps there is another approach to SETI that avoids many of the challenges of looking for physical artifacts and the limitation of the possibility of a small L in the Drake equation. Maybe we can look for “electromagnetic artifacts.” Electromagnetic artifacts can be thought of as “virtual” Von Neumann probes where instead of having physical devices replicate and propel themselves through the galaxy, electromagnetic signals are initially transmitted where their signaling properties are designed to be amplified and replicated using natural stellar and physical processes. Such natural processes might include gravitational lensing to refocus signals and using stellar lasers or masers to amplify a given signal.
Such self amplifying and replicating electromagnetic signals are different than normal transmissions used in beacons in that they are not intended to be point to point communications. Like Von Neumann probes they are expected to randomly propagate through a galaxy using passing stellar systems to amplify and replicate the original transmission. This capability might allow electromagnetic Von Neumann probes to propagate throughout a galaxy much faster than physical probes. The advantage of a low cost self amplifying and replicating signal is that you only need one replicant signal in a billion or trillion to multihop many hundreds of stars and be detected by another civilization.
Gravitational lensing has been used in astronomy for some time. In an interesting post (https://centauri-dreams.org/?p=10123), Claudio Maccone calculates that by using gravitational lens and with satellites at the appropriate focal points of each solar system a detectable radio signal could be sent from our solar system to Alpha Centauri that uses less than 10-4 watts, i.e. one tenth of a milliwatt!!
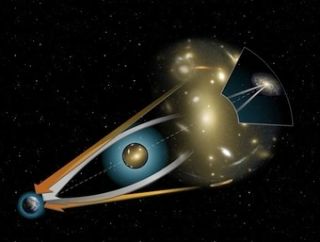
Normally a signal sent from earth would not benefit from gravitational lensing as the focal point would be too far out (past Pluto). The signal might be bent but otherwise it will quickly suffer dispersion like any other signal. To achieving lensing a signal must pass both sides of the sun at roughly the same time and the wavefront recombine coherently on the far side.
One solution would be to put satellites at the focal point as Moccone has suggested. But an easier earthbound solution is to use earth-bound phased array antenna. A signal generated by a phased array could be made with a wavefront that looks like it originated from the Sun’s focal point or even a more distant point. You may want to use a more distant (or closer) artificial focal point in order to use gravitational lens of a more distant star; i.e launch our signal so that it is deliberately out of focus (but collimated) by our sun but comes into focus at a distant star. These are the same principles used in multi-lens cameras or telescopes. The converging point of the signal could be at the focal point of a distant star or perhaps even a multi-hop star.
Once you have a collimated signal with a coherent wavefront and wide aperture (i.e. the Sun’s diameter) you could in theory hop many stars that are in line with our orbital plane (or will be by the time the signal gets there). You could also steer the signal up and down a little bit from the orbital plane with a phased array antenna.
Additional amplification could use natural masers/lasers in our sun or distant star. There are several suitable natural maser frequencies – the choice of appropriate frequency will depend on the types of stars we are aiming at.
Stellar masers have been known for some time. A maser emission may be created in molecular clouds, comets, planetary atmospheres, and stellar atmospheres. They are frequently used in radio astronomy as they provide important information on distant stellar objects, such as temperature, velocity, etc. The first “natural” laser in space was detected by scientists on board NASA’s Kuiper Airborne Observatory (KAO) in 1995 as they trained the aircraft’s infrared telescope on a young, very hot, luminous star in the constellation Cygnus. Since then many other examples of both planetary and stellar lasers have been found.
The problem with natural masers/lasers is their noise level. The same is true of gravitational lensing if the signal passes through, or close to the corona. To extract the signal one would need a reference clock – and this would be the tell tale signal that it is artificial. I would theorize a good reference clock would be a distant highly regular timed quasar.
In effect we have created a beacon, but rather than looking in the water hole, a receiving civilization would have to look at the known natural maser/laser frequencies and then auto-correlate the signal to see if they can extract a reference clock. This is the same technology we use in very long baseline interferometry used in deep space radio dishes.
Now the interesting thing about natural masers/lasers is that they can amplify a given signal not only in the line of propagation but in other orthogonal directions as well. If the signal maintains its coherent wavefront ( still needs to be verified) then a given signal can be replicated in many directions from a given star. If it is still collimated then it would also look like a beacon pointed in some unknown random direction. A single star could produce many beacons like a disco ball based on the original transmission.
On a small scale, real world examples of self amplifying and replicating electromagnetic signals already exist. They are called Long Delayed Echoes (LDEs). They were first discovered in 1927 by amateur radio enthusiasts who noticed echoes of their original radio transmissions delayed by up to 40 seconds.
Up to now I have only been talking about signalling from our limited knowledge. I suspect there are other stellar phonemena, like with long delay echoes, that could be used to replicate and amplify signals.
There is no clear agreement on what causes LDEs, but there are several hypotheses on some possible natural phenomena that may enable electromagnetic echoes. These include such things as reflections from distant plasma clouds originating from the sun, magnetosphere ducting, mode conversion and four wave mixing, etc. While these natural phenomena may not be suitable processes for interstellar electromagnetic transmission they do demonstrate the possibility that perhaps equivalent stellar processes could be used on a larger scale to amplify and replicate electromagnetic signals much greater distances.
Given that they depend on natural physical processes for amplification and replication, the originating transmission will likely not need to be that powerful or directional. It is conceivable that low power transmissions are that all is required to launch a self amplifying and replicating electromagnetic probe. Most importantly, with electromagnetic replication a single instance of the signal may be replicated thousands or millions of times as it propagates through the galaxy or the universe. Compared with physical probes replication could accelerate on an exponential scale increasing the probability of detection, particularly in a ‘rare Earth’ situation.
Issues to Be Surmounted
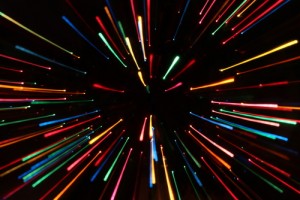
Although using self amplification and replication sounds like an interesting idea there are a number of theoretical and physical challenges that still must be addressed. How, for example, to account for the proper motion of our sun versus distant stars? Will any such signal just sweep by like a beam from a lighthouse and make detection near impossible (e.g., the ‘Wow’ signal?) Other issues include accuracy and phase noise in phased array antenna – how precisely can we control a given signal?
Thermal noise in stellar atmospheres that are to be used for laser/maser gain is clearly a major issue. The “gain” of a stellar laser or maser is also very limited as there is no resonant cavity. There are also a host of well known problems with current inter-stellar electromagnetic signaling such as attenuation, dispersion, group delay, etc etc. In addition, the proper motion of our solar system and that of any intermediate amplifying and replicating stellar system would seem to make detection difficult.
To address these limitations in detecting such a signal it would be useful to explore how we might deploy a self replicating electromagnetic signal given our current technology limitations. Many techniques currently being used in modern radio and optical communication systems could be deployed to launch a self replicating and amplifying electromagnetic probe.
Clearly an external reference clock or coding reference would be required to extract any signal that was amplified by a stellar laser/maser as the inherent stellar noise would mask any external signal. A quasar may provide such a reference signal. Phased array antennae and signal preconditioning could be used to take advantage of gravitational lensing without placing transmitters at the lens focal point.
Gravitational lenses also act as gradient amplifiers and with time delay from two phased array sources it might be possible to regenerate a given signal (such as timing, shaping etc) using all electromagnetic techniques – a process now largely done by electronics. By constantly steering the phased array transmitter(s) a signal could be directed like a beacon at nearby stars that are aligned with our orbital plane to take advantage of the sun’s gravitational lens. Similarly steering of the phased array might allow a given signal to converge at the gravitational focal point of a nearby star where it could be amplified and replicated by that star to be propagated to even more distant stars.
With a little imagination and speculation on the future direction of these technologies a self amplifying and replicating electromagnetic Von Neumann probe might be within our technology capability. Once we have identified a plausible approach on how we would deploy such signals, the obvious next step would be to see if we can detect such signals.
Conclusion
Up to now we have always assumed that a distant civilization would want to send a direct beam at us and so we have been exploring that part of the electromagnetic spectrum that has the least absorption and attenuation. But if a distant civilization discovered it could use natural low cost processes to amplify and replicate a signal that would be its preferred route, especially if intelligence is a rarity in our galaxy. With laser/maser replication millions or billions of signals could be traversing the galaxy, of which only one needs to be detected by another civilization. This would be a much cheaper approach from an energy perspective than building omni-directional antennas, Dyson spheres, or aiming a beacon at our Sun, etc.
The assumption here is that a distant civilization wants to make contact with us, but I suspect that self replicating and amplifying signals will be transmitted for much more mundane reasons. If a self amplifying and replicating signal can be transmitted practically forever, really cheaply, then forget about contacting other civilizations, I want knowledge of my brief presence here on earth to be preserved forever. Paradoxically, religion and belief in the hereafter may be a driving force to transmit such signals!
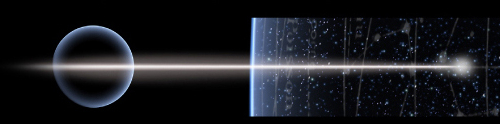
by Paul Gilster | Jan 27, 2017 | Culture and Society |
When we contemplate contact scenarios between ourselves and extraterrestrial civilizations, we can profit from remembering our own history. The European arrival in the Americas is often a model, but there are other events of equal complexity. In the essay below, Michael Michaud looks at America’s encounter with Japan to examine how we might react to a civilization not vastly more advanced in technology than our own. A familiar figure on Centauri Dreams, Michaud is now retired from an extensive diplomatic career that took him from director of the U.S. State Department’s Office of Advanced Technology to chairman of working groups at the International Academy of Astronautics that discuss SETI issues, along with posts as Counselor for Science, Technology and Environment at U.S. embassies in Paris and Tokyo. He is also the author of the seminal Contact with Alien Civilizations (Springer, 2007).
By Michael A.G. Michaud
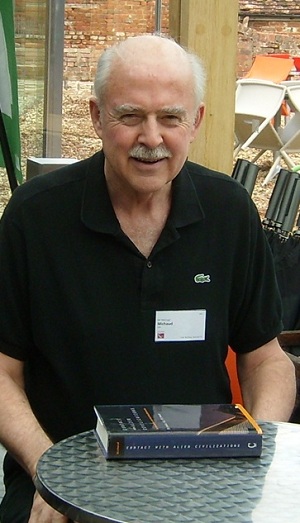
In the literature about possible future contact with an extraterrestrial civilization, one of the most familiar presumptions is that the aliens will employ technologies vastly more advanced and more powerful than our own. Hollywood has given us binary images of such technologically empowered beings, depicting them as either benign altruistic teachers or as monsters who want to destroy us. There is a lot of room between those extremes.
A countervailing theory suggests that we are most likely to encounter a technological civilization closer to our own level, as the most advanced would ignore us or treat us as irrelevant. What might happen if we came into direct contact with a civilization whose technologies were only a century in advance of ours? Here is an Earthly example.
In 1852, U.S. President Millard Fillmore assigned U.S. Navy Commodore Matthew Perry to force the opening of Japanese ports to American trade. Perry was to deliver a letter from the President to the Emperor of Japan.
At the time, the only authorized port of entry into Japan was Nagasaki, where the Dutch maintained a trading post. The Japanese, forewarned by the Dutch that Perry’s ships were on their way from the United States, refused to change their 220 year old policy of exclusion.
Perry’s mission to Japan was part of a much longer voyage around southern Africa to Asia, a showing of the American flag. After an eight month journey with multiple port calls, Perry’s squadron of four ships reached the entry to Edo (now Tokyo) Bay on July 8, 1853.
Numerous Japanese fishing boats hastily retreated from the American ships, whose crews were at battle stations. Perry’s account reports that the fishermen seemed astonished to see the steam powered American vessels proceed against the wind with their sails furled.
Japanese officials in boats approached the American ships several times, asking them to leave. The first boat bore large banners with characters inscribed on them. The Americans, who could not read Japanese, conjectured that this boat was a government vessel of some kind.
Another Japanese boat approaching the American ships carried a man holding up a scroll which he read aloud. He was admitted aboard to meet with a lower-ranking American officer. The scroll, later found to be a document in French, conveyed an order that the American ships should leave, reiterating that Nagasaki was the only place in Japan for trading with foreigners.
The crews of Japanese “guard boats” made several attempts to board Perry’s ships, but were repelled by Americans with pikes, cutlasses, and pistols. No casualties were reported.
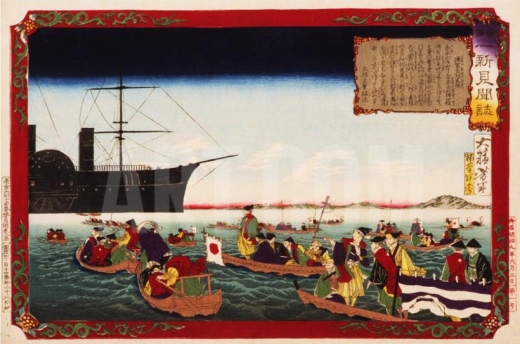
Image: American Navy Commodore Matthew Perry arrives in Japan, August 7, 1853. Credit: Tsukioka Yoshitoshi, woodblock print.
Through his officers, Perry warned that he would not permit Japanese guard boats to remain close to his ships. If they were not immediately removed, he would disperse them by force. When a few guard boats remained, Perry sent armed men to drive them away.
The Japanese made token shows of force on shore, firing out of date cannons and launching rockets. None seemed to be aimed at the American ships.
Perry warned the Japanese that, if they chose to fight, he would destroy them. In a demonstration of force that did no physical harm, he ordered blank shots to be fired from his squadron’s 73 cannons.
The Americans discovered that Japanese defenses were more for show than combat. Using a telescope to observe forts on headlands, they found some in an unfinished state. Screens had been stretched in front of the breastworks, possibly with the intention of making “a false show of concealed force.” The narrative’s writer observed that the Japanese had not calculated on the “exactness of view” afforded by a telescope.
Perry strove to impress the Japanese with “a just idea” of the power and superiority of the United States. He described his demands as “a right,” and not an attempt to solicit a favor. He expected “those acts of courtesy which are due from one civilized nation to another.” If the Japanese assumed superiority, that was a game he could play as well as they.
Perry refused to meet with lower level Japanese officials. His narrative observes that the more exclusive he made himself, and the more unyielding he was, the more respect “these people of forms and ceremonies” would award him.
A Japanese man who was described as a Governor came to Perry’s flagship, where he met with American officers while Perry remained invisible. (The visitor actually was the Deputy Governor.) At one point, lower ranking American officers dealing with the Japanese elevated Perry’s rank from Commodore to Admiral.
As one might expect, language was a problem. The Americans had one interpreter who knew Chinese and another who knew Dutch, but no one who spoke Japanese. The Japanese provided an interpreter who spoke Dutch; the two sides used a third country’s language.
The Americans warned that if the Japanese did not appoint a suitable person to receive the President’s letter and other documents from the American capital, Perry’s forces would go ashore with sufficient force and deliver them in person (by implication, to the Emperor). They pointed out that that one hour’s steaming would bring Perry’s ships in sight of Edo (Tokyo). Perry did send one of his ships closer to Edo, anticipating that this would alarm the Japanese authorities and induce them to give a more favorable answer to his demands.
When Perry sent out boats to survey the coastline, Japanese vessels carrying armed men rushed toward them. The American officer in charge of the surveying party gave orders for his men to arm their weapons. Seeing the armed sailors, the Japanese avoided a direct confrontation.
At last, the real Governor visited Perry’s ship, exhibiting a letter from the Emperor that met Perry’s demand for a high level Japanese official to accept the letter from the American President. The Governor provided the Americans with a copy in Dutch.
Arrangements were made for a ceremony on shore. Japanese officials asked Perry to move his ships close to a beach in modern day Yokosuka (where there is now an American naval base). There he would be allowed to land.
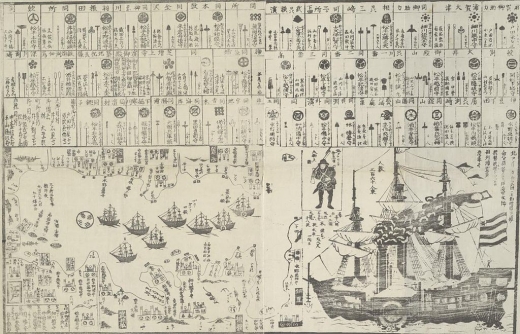
Image: Japanese 1854 print describing Commodore Matthew Perry’s “Black Ships.” Source: Wikimedia Commons.
Perry’s approach the next morning was announced by American guns. In a formal ceremony under a large, decorated tent, he presented the documents from Washington. He promised to return the following year to receive the Japanese reply.
Perry commented in his report that the Japanese officials showed a quiet dignity of manner and never lost their self-possession. However backward the Japanese might be in practical science, he wrote, the best educated among them were “tolerably well-informed of progress among more civilized nations.” Perry expressed a hope in his account that “our attempt to bring a singular and isolated people into the family of civilized nations may succeed without resort to bloodshed.”
Perry returned to Japan in February 1854 with ten ships and 1,600 men, putting even more pressure on the Japanese. After initial resistance, he was permitted to land at Kanagawa, near present-day Yokohama. A month of negotiations led to the first Convention between Japan and the United States.
The American negotiator on the spot had greater latitude in that pre-radio era, when communication with capitals was slow. Unfortunately, Perry was mistaken in his belief that this agreement had been made with the Emperor’s representatives. He did not understand the position of the Shogun, the de facto ruler of Japan.
What lessons can we draw from this? Most obvious is that finding a shared language would be difficult. Points might be made through actions or images rather than words.
Another lesson is that this meeting of technological unequals did not lead to armed conflict. The Americans used their superior weapons and propulsion technologies to intimidate, not to damage or conquer. Despite their threats, they acted with restraint, and got their way without violence.
The Japanese, though making only weak shows of force, insisted on being treated as diplomatic equals through rituals and symbols. The Americans, as the more powerful civilization, maintained a balance between intimidation and respect.
We may want to keep histories like this in mind as we weigh possible contact scenarios. Contact may not be between cultures separated by millennia of scientific and technological development.
Readers interested in a complete account of this event may wish to look at Commodore M.C. Perry, Narrative of the Expedition to the China Seas and Japan, 1852-1854, reprinted by Dover in 2000. As Perry’s visit took place in the pre-photographic age, that report was illustrated with lithographs and woodcuts by American on-board artists.
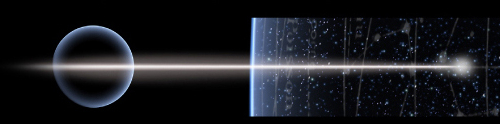
by Paul Gilster | Jan 26, 2017 | Exoplanetary Science |
A key way to learn more about a given exoplanet is to home in on the properties of its star. So argue Stephen Kane (San Francisco State University) and colleagues in a new paper slated for the Astrophysical Journal. The star in question is Wolf 1061 (V2306 Ophiuchi), an M-class red dwarf some 13.8 light years away in the constellation Ophiuchus. In December of 2015, Australian astronomers announced the discovery of three planets around the star.
Drawn out of data from the HARPS spectrograph at La Silla, the planets are all super-Earths, their radial velocity data supplemented with eight years of photometry from the All Sky Automated Survey. All three seem likely to be rocky planets, but firming this up would take transits, which the discovery team at the University of New South Wales estimated might occur, with a likelihood of about 14 percent for the inner world, dropping to 3% for the outer.
Kane and team investigate the transit question in light of the fact that that two recent papers have produced sharply different orbital periods for the outermost planet here, though both find planet c near or within the habitable zone, which itself depends on the star’s luminosity and effective temperature. Both the recent papers see reasonably high transit possibilities, but Kane’s work rules out transits of the two inner worlds, leaving open a possibility for the outer.
The researchers have used observations from the Center for High Angular Resolution Astronomy (CHARA) interferometric array at Mount Wilson Observatory near Los Angeles. From this emerges a precise stellar radius measurement (0.3207±0.0088 R?), from which the team has calculated the star’s effective temperature and luminosity. The photometry data reveal a stellar rotation period of 89.3±1.8 days. The work has useful implications for upcoming space-based exoplanet studies. As the paper notes:
The assessment of host star properties is a critical component of exoplanetary studies, at least for the realm of indirect detections through which exoplanet discoveries thus far have predominantly occurred. This situation will remain true for the coming years during which the transit method will primarily be used from space missions such as the Transiting Exoplanet Survey Satellite (TESS), the CHaracterising ExOPlanet Satellite (CHEOPS), and the PLAnetary Transits and Oscillations of stars (PLATO) mission. Of particular interest are the radius and effective temperature of the stars since the radius impacts the interpretation of observed transit events and the combination of radius and temperature is used to calculate the extent of the HZ.
And indeed, it is through this painstaking analysis of stellar properties that the researchers have been able to calculate habitable zone boundaries for Wolf 1061 of 0.09–0.23 AU. Have a look at the paper’s Figure 8, which shows the Wolf 1061 system as diagrammed from above.
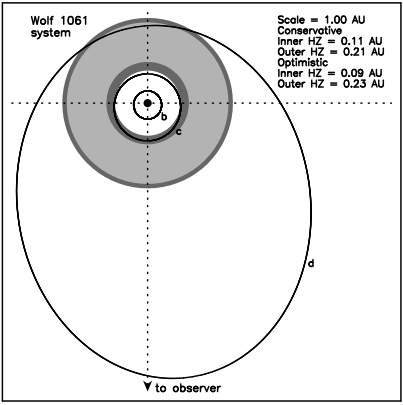
Image: Figure 8 from the paper shows the orbits of the planets overlaid on the habitable zone. The scale here is 1.0 AU to the side, with the ‘conservative’ habitable zone shown as light gray, and the more optimistic extension shown in dark gray. Credit: Kane et al.
Notice the outer planet (d), which passes briefly through the habitable zone at closest approach to the star before widening further out in its eccentric orbit. Indeed, only 6 percent of the orbital period takes place within the habitable zone. Planet c spends 61 percent of its orbit within the habitable zone, but only within the optimistic assumptions for the HZ. Taking into account the recent orbital solutions for this system, both inner worlds are problematic:
…planet c is quite similar to the case of Kepler-69 c, which was proposed to be a strong super-Venus candidate by Kane et al. (2013). Indeed, both of the inner two planets, terrestrial in nature according to the results of both Wright et al. (2016) and Astudillo-Defru et al. (2016b), lie within the Venus Zone of the host star (Kane et al. 2014) and are thus possible runaway greenhouse candidates.
Measuring stellar parameters unlocks the boundaries of the habitable zone, allowing the researchers to study the planetary orbits and weigh the chances for liquid water on the surface. The results hardly favor the Wolf 1061 system as a promising candidate for life.
We find that, although the eccentric solution for planet c allows it to enter the optimistic HZ, the two inner planets are consistent with possible super-Venus planets (Kane et al. 2013, 2014). Long-term stability analysis shows that the system is stable in the current configuration, and that the eccentricity of the two inner planets frequently reduces to zero, at which times the orbit of planet c is entirely interior to the optimistic HZ. We thus conclude that the system is unlikely to host planets with surface liquid water.
The paper is Kane et al., “Characterization of the Wolf 1061 Planetary System,” accepted for publication at the Astrophysical Journal (preprint).
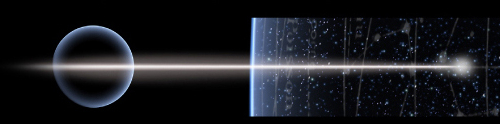
by Paul Gilster | Jan 25, 2017 | Outer Solar System |
The Japanese PROCYON spacecraft (Proximate Object Close flyby with Optical Navigation) has just given us an interesting case of repurposing a scientific instrument, not to mention drawing value out of a mission whose initial plans had gone awry. Launched together with JAXA’s Hayabusa 2 probe in late December of 2014, PROCYON was to have flown by asteroid 2000 DP107 in 2016, but a malfunctioning ion thruster put an end to that plan.
Fortunately, PROCYON carried LAICA, a telescope that was put to use to study the Earth’s geocorona (the outermost layer of the atmosphere). Developed at Japan’s Rikkyo University, LAICA observes emissions from hydrogen atoms, a useful capability when turned to comet studies, as a team of researchers has now done with comet 67P/Churyumov-Gerasimenko. Water being the most abundant cometary ice, its release rate helps map activity on the comet and offers clues to how water was incorporated into comets in the early Solar System.
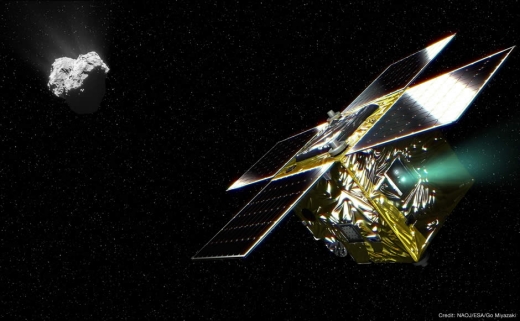
Image: The PROCYON spacecraft and comet 67P/Churumov-Gerasimenko (Conceptual Image). Credit: NAOJ/ESA/Go Miyazaki.
The researchers, from the National Astronomical Observatory of Japan, University of Michigan, Kyoto Sangyo University, Rikkyo University and the University of Tokyo, set out to map the entire hydrogen coma of comet 67P, the same comet studied in such spectacular detail by the European Space Agency’s Rosetta mission. The challenge to Rosetta, however, was that being inside the cometary coma, it could not observe the entire coma structure.
None of this was in the original PROCYON mission, but it became clear that the spacecraft could be valuable in supplementing the Rosetta observations. Rosetta had studied specific areas on the comet, but the researchers wanted an estimate of the total amount of water released by the comet per second, which in turn demanded a model for the coma itself. If Rosetta could not provide the answer, PROCYON could study the coma in its entirety.
Adapting PROCYON/LAICA for a comet proved workable. The hydrogen atoms in a cometary coma come from water molecules ejected from the nucleus which are then broken apart by ultraviolet radiation from the Sun. Coma models based on these processes allowed the team to estimate the water release rate based on a brightness map of the hydrogen atoms.
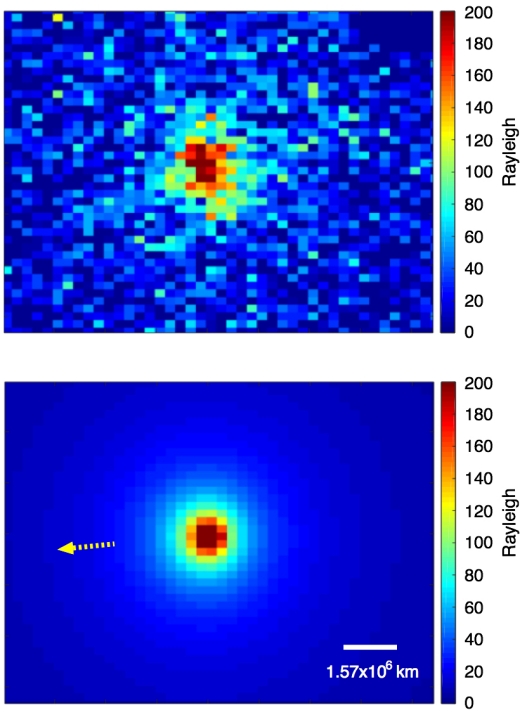
Image: Processed and cropped hydrogen-Lyα image of the comet 67P/C-G in Rayleigh units (upper panel) taken by the LAICA telescope on September 13, 2015 UT and the hydrogen coma appearance predicted by a two-dimensional axi-symmetric model of the atomic hydrogen coma (lower panel). The yellow dotted arrow in the lower panel indicates the direction to the Sun at the time of observation. Credit: NAOJ.
Working with observations of comet 67P’s entire hydrogen coma, the researchers were able to derive its absolute water production rates near the 2015 perihelion. This allowed them to test their developing models for the coma, which could be combined with the Rosetta results to estimate the total ejected mass of the comet during this period. Credit the high spatial resolution of the LAICA instrument and the pointing control of PROCYON for the result.
These are useful results, but let’s also look at what they imply about how we design our missions. PROCYON is a small cube about 60 cm to the side weighing 65 kg. The low-cost mission has been able to support key elements of a much larger mission with additional observations in a way that JAXA believes to be a model for small spacecraft in the future.
As we continue to explore what we can do with CubeSats and other micro-designs, we can think in terms of ‘clusters’ of small spacecraft networking together to reduce overall risk and provide widely dispersed observing platforms for a variety of targets. Swarms of micro-sailcraft to the outer planets are just one scenario that may grow out of spacecraft interactions like these.
The paper is Shinnaka et al. 2017 “Imaging observations of the hydrogen coma of comet 67P/Churyumov-Gerasimenko in September 2015 by the PROCYON/LAICA,” Astronomical Journal, Volume 153, Issue 2, Article number 76 (24 January 2017). Abstract.
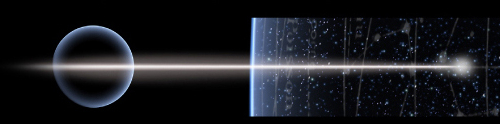
by Paul Gilster | Jan 24, 2017 | Outer Solar System |
It doesn’t stretch credulity to hypothesize that the early Earth benefited from an influx of comet and asteroid material that contributed water and organic compounds to its composition. The surface of a world can clearly be affected by materials from other bodies in the Solar System. Now we’re learning that the dwarf planet Ceres may have a surface dusted by material from asteroid impacts. The findings come from a team of astronomers investigating Ceres with SOFIA, the airborne Stratospheric Observatory for Infrared Astronomy. The observatory is a highly modified 747SP aircraft carrying a 2.5m reflecting telescope.
The study shows that not just Ceres but other asteroids and dwarf planets may be coated with asteroid fragments, a result that adjusts our view of Ceres’ surface composition. After all, what we’re looking at may simply be the result of asteroid impacts in the early days of the Solar System’s formation. Three quarters of all asteroids, including Ceres, have been classified as type C (carbonaceous) on the basis of their colors, but the SOFIA infrared data show a substantial difference between the dwarf planet and C-type asteroids in nearby orbits.
Carbonaceous asteroids are dark (albedo in the range of 0.03-0.09, on a scale where a white, perfectly reflecting surface has an albedo of 1.0), with a composition depleted in hydrogen, helium and other volatiles. What SOFIA shows us is that Ceres doesn’t fit this model. Pierre Vernazza is a research scientist at the Laboratoire d’Astrophysique de Marseille:
“By analyzing the spectral properties of Ceres we have detected a layer of fine particles of a dry silicate called pyroxene. Models of Ceres based on data collected by NASA’s Dawn as well as ground-based telescopes indicated substantial amounts of water-bearing minerals such as clays and carbonates. Only the mid-infrared observations made using SOFIA were able to show that both types of material are present on the surface of Ceres.”
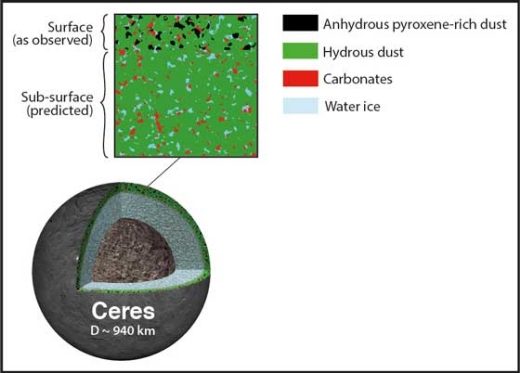
Image: Ceres’ surface is contaminated by a significant amount of dry material while the area below the crust contains essentially water-bearing materials. The mid-infrared observations revealed the presence of dry pyroxene on the surface probably coming from interplanetary dust particles. The Internal structure of the Dwarf Planet Ceres was derived from NASA Dawn spacecraft data. Credit: SETI Institute.
Interplanetary dust particles, according to this SETI Institute news release, are the most likely source for the pyroxene, and are also implicated as having accumulated on other asteroid surfaces. Ceres thus takes on the coloration of some of its drier neighbors, while actually housing more substantial resources of water below. The larger picture is that infrared observations may help us better understand an asteroid’s true composition. Vernazza even speculates that ammoniated clays mixing with watery clay on Ceres may point to an origin in the outer parts of the Solar System, with migration occurring later in the dwarf planet’s life.
“The bottom line is that seeing is not believing when it comes to asteroids,” says Franck Marchis, senior planetary astronomer at the SETI Institute, a researcher who collaborated in this project. “We shouldn’t judge these objects by their covers, as it were.”
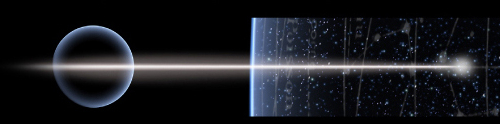