by Paul Gilster | Feb 28, 2018 | Exoplanetary Science |
What is it we are looking for when we probe nearby planetary systems? Certainly the search for life elsewhere compels us to find planets like our own around stars much like the Sun. But surely our goal isn’t restricted to finding duplicate Earths, if indeed they exist. A larger goal would be to find life on planets unlike the Earth — perhaps around stars much different from the Sun — which would give us some idea how common living systems are in the galaxy.
And beyond that? The ultimate goal is simply to find out what is out there. That takes in outcomes as different as widespread microbial life, perhaps leading to more complex forms, and barren worlds in which life never emerged. A galaxy filled with life vs. a galaxy in which life is rare offers us two striking outcomes. We ignore preconceptions to find out which is true.
Flaring Red Stars
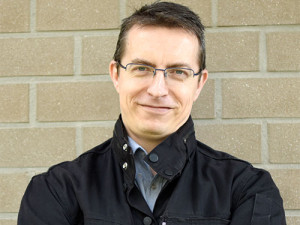
Let’s try to put Proxima Centauri’s recent flare, discussed yesterday, in context. Events like this highlight our doubts about the viability of red dwarf systems in developing life. Flares are not uncommon on ultra-cool dwarf stars, meaning nearby planets in any habitable zone (as defined by liquid water at the surface) would be bathed in ultraviolet and X-rays. We face the distinct possibility that such planets might have never become habitable in the first place, their oceans vaporized, their atmospheres hopelessly compromised.
I’m interested to see how Amaury Triaud and Michaël Gillon see things. It was their team at the University of Liège in Belgium and the University of Cambridge that did the initial work on TRAPPIST-1, a red dwarf around which ultimately seven planets were found to orbit. As the scientists point out in a recent essay for Big Think, three of the planets here are intriguing from the standpoint of habitability, and we’ve recently seen new work on their potential atmospheres.
Image: Exoplanet hunter Michaël Gillon (University of Liège, Belgium).
This is what is so exciting about red dwarfs. While we continue to look for rocky worlds around G-class stars, we’re putting the tools for studying red dwarf planets to use right now. Consider what we’ve learned already: TRAPPIST-1 d, e and f do not show signs of puffy atmospheres rich in hydrogen, which goes a long way toward eliminating them as Neptune-like worlds, and leaves open the issue of more compact atmospheres that could sustain life. We’ve also had a fine-tuning of mass estimates (see TRAPPIST-1: Planets Likely Rich in Volatiles). Thus a star producing a scant 0.05 percent as much light as the Sun is beginning to yield its secrets.
Triaud and Gillon look at the path forward, pointing to atmospheric work with the James Webb Space Telescope. It’s clear they have little patience with those content to rule out habitability without a great deal of further evidence. The arguments they examine are familiar: Planets around such stars may be tidally locked, and indeed, in systems like TRAPPIST-1, so tightly packed that they would produce major instabilities. They acknowledge the flare problem Proxima Centauri has so vividly demonstrated.
But what we are dealing with is a set of unknowns, and the exciting thing is that we are closing in on the ability to produce answers to many of these objections. From the essay:
Far from holding us back, those arguments motivated us. Now we can assess the actual conditions, and explore counter-arguments that Earth-sized planets around stars such as TRAPPIST-1 might in fact be hospitable to life. Oceans and thick atmospheres could mitigate the temperature contrast between day and night sides. Tidal interaction between close-orbiting planets might provide energy for biology. Some models suggest that planets forming around ultra-cool dwarfs start out with much more water than Earth has. Ultraviolet radiation could help to produce biologically relevant compounds… We are optimistic.
It’s the nature of that optimism that we need to explore. For Triaud and Gillon are not overlooking the problems of astrobiology here to insist on a result they want to see. Instead, they’re pointing to a demonstrable fact: In our rush to find a ‘twin’ of the Earth, we sometimes forget that our planet is not necessarily the template for life elsewhere. Indeed, searching for an Earth twin is a highly conservative approach. “Research should be about finding what we don’t already know,” they argue, and in the case of exoplanets, that certainly encompasses the nature of the 75 percent of the galaxy comprised of red dwarfs down to tiny ultra-cool stars.
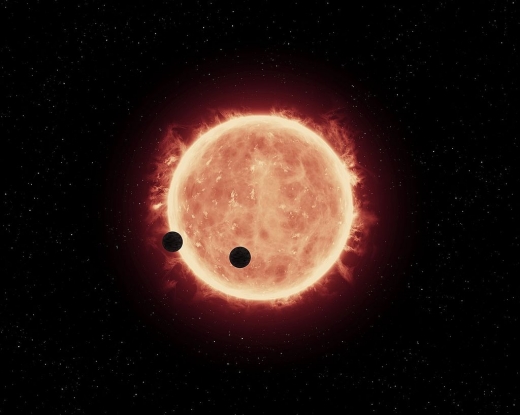
Image: This artist’s impression shows two Earth-sized worlds passing in front of their parent red dwarf star, which is much smaller and cooler than our Sun. Astronomers using the Hubble instrument recently used transmission spectroscopy, in which starlight passes through potential atmospheres of planets, to look for evidence of extended hydrogen atmospheres around several TRAPPIST-1 planets. The lack of such atmospheres makes it unlikely that these worlds are Neptune-like. Image credit: ESA/Hubble.
If we’re looking for life elsewhere in the universe, we have only to consider the range of planetary systems thus far discovered to know that we need to study systems utterly unlike our own. The goal then, as the authors put it, is to measure the total frequency of biology. That makes red dwarfs an obvious target, particularly when, like TRAPPIST-1, nearby examples offer such deep transits, so much more amenable to current study than Earth-sized worlds transiting G-class stars like our Sun. Indeed, a TRAPPIST-1 transit can be 80 times more prominent, with like gains in the visibility of atmospheric chemistry.
So while we continue to search for a true Earth analog around a star like the Sun, we can put nearby red dwarf planets under immediate investigation, with TRAPPIST-1 transits happening in a matter of days or weeks rather than once a year. The nature of their atmospheres is the first priority, helping us decide what surface conditions may be like. The search for biosignatures of biologically produced gases follows, with the added advantage at TRAPPIST-1 in having so many planets that can be directly compared to each other.
The TRAPPIST (Transiting Planets and Planetesimals Small Telescopes) facility we used to find the TRAPPIST-1 planets was just the prototype of a more ambitious planet survey called SPECULOOS (Search for habitable Planets Eclipsing Ultra-Cool Stars), which has already begun operations. We expect to find many more Earth-sized, rocky planets around dwarf stars within the next five years. With this sample in hand, we will explore the many climates of such worlds. The solar system contains two: Venus and Earth. How many different types of environments will we discover?
If red dwarf planets near us are shown to be devoid of life, we may eventually learn that life is rarer than we thought. But I return to my earlier sentiment — at heart, our goal in studying the universe isn’t to find life, but rather to find what is out there. If we were to learn that life is vanishingly rare, that would be a finding of immense significance for our stewardship of our own planet, teaching us how unusual it may in fact be. In any case, while we all have ideas about what we hope to find, the universe will surely keep forcing us to adjust our expectations.
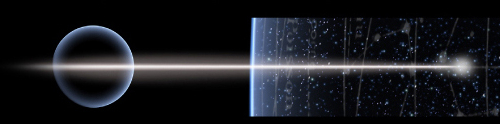
by Paul Gilster | Feb 27, 2018 | Exoplanetary Science |
News of a major stellar flare from Proxima Centauri is interesting because flares like these are problematic for habitability. Moreover, this one may tell us something about the nature of the planetary system around this star, making us rethink previous evidence for dust belts there.
But back to the habitability question. Can red dwarf stars sustain life in a habitable zone much closer to the primary than in our own Solar System, when they are subject to such violent outbursts? What we learn in a new paper from Meredith MacGregor and Alycia Weinberger (Carnegie Institution for Science) is that the flare at its peak on March 24, 2017 was 10 times brighter than the largest flares our G-class Sun produces at similar wavelengths (1.3 mm).
Image: The brightness of Proxima Centauri as observed by ALMA over the two minutes of the event on March 24, 2017. The massive stellar flare is shown in red, with the smaller earlier flare in orange, and the enhanced emission surrounding the flare that could mimic a disk in blue. At its peak, the flare increased Proxima Centauri’s brightness by 1,000 times. The shaded area represents uncertainty. Credit: Meredith MacGregor.
Lasting less than two minutes, the flare was preceded by a smaller flare, as shown above, revealing the interactions of accelerated electrons with Proxima Centauri’s charged plasma. We already knew that Proxima produced regular X-ray flares (recent studies have pegged the rate at one large event every few days), though these are much smaller than the flare just observed. The effects of such flaring on Proxima b could be profound, according to MacGregor:
“It’s likely that Proxima b was blasted by high energy radiation during this flare. Over the billions of years since Proxima b formed, flares like this one could have evaporated any atmosphere or ocean and sterilized the surface, suggesting that habitability may involve more than just being the right distance from the host star to have liquid water.”
The issue has significance far beyond Proxima because M-class stars are the most common in the galaxy. They’re also given to pre-main sequence periods marked by frequent changes in luminosity, and prone to a high degree of stellar activity throughout their lifetimes. Proxima Centauri, spectral class M5.5V, has long been known to be a flare star, leading to the current interest in determining the effects of its variability on the single known planet.
MacGregor and Weinberger worked with data from the ALMA 12-m array and the Atacama Compact Array (ACA). The datasets from these observations were examined by Guillem Anglada (Instituto de Astrofísica de Andalucía, Granada, Spain) in 2017, whose team found signs of a dust belt of about 1/100th of Earth’s mass in the 1-4 AU range, with the possibility of another outer belt. These two structures seemed to parallel the asteroid and Kuiper belts we find in our own Solar System, and it was thought that the inner belt might help us constrain the inclination of the Proxima Centauri system, while giving us an idea of its complexity.
I should pause to note that Guillem Anglada is not Guillem Anglada-Escudé, who led the work that discovered Proxima b — the similarity in names is striking but a coincidence. Making this even more confusing is the fact that Guillem Anglada-Escudé is a co-author on the dust paper on which Guillem Anglada was lead author. If we can get the names straight, we can go on to note that the MacGregor/Weinerger results question whether the dust belts are really there.
For MacGregor and Weinberger looked at the ALMA data as a function of observing time, noticing the transient nature of the radiation from the star. From the paper:
The quiescent emission detected by the sensitive 12-m array observations lies below the detection threshold of the ACA observations, and the only ACA detection of Proxima Centauri is during a series of small flares followed by a stronger flare of ? 1 minute duration. Due to the clear transient nature of this event, we conclude that there is no need to invoke the presence of an inner dust belt at 1 ? 4 AU. It is also likely that the slight excess above the expected photosphere observed in the 12-m observations is due to coronal heating from continual smaller flares, as is seen for AU Mic, another active M dwarf that hosts a well-resolved debris disk. If that is the case, then the need to include warm dust emission at ? 0.4 AU is removed. Although the detection of a flare does not immediately impact the claim of an outer belt at ? 30 AU, the significant number of background sources expected in the image and known high level of background cirrus suggest that caution should be used in over-interpreting this marginal result.
So we still have the possibility of an outer belt, though not one that can be claimed with any degree of certainty, and we have removed the need for the inner belt. Proxima Centauri may indeed have other planets and a more complex system than we currently know, but the data from ALMA and ACA now appear to be indicative only of the known flaring phenomenon.
“There is now no reason to think that there is a substantial amount of dust around Proxima Cen,” says Weinberger. “Nor is there any information yet that indicates the star has a rich planetary system like ours.”
The paper is MacGregor et al., “Detection of a Millimeter Flare From Proxima Centauri,” accepted at Astrophysical Journal Letters (preprint). The paper on dust belts is Anglada et al., “ALMA Discovery of Dust Belts Around Proxima Centauri,” accepted at Astrophysical Journal Letters (preprint).
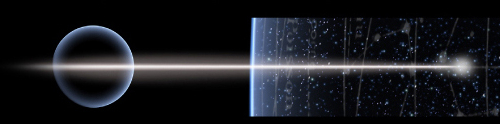
by Paul Gilster | Feb 23, 2018 | Exoplanetary Science |
At the last Tennessee Valley Interstellar Workshop, I was part of a session on biosignatures in exoplanet atmospheres that highlighted how careful we have to be before declaring we have found life. Given that, as Alex Tolley points out below, our own planet has been in its current state of oxygenation for a scant 12 percent of its existence, shouldn’t our methods include life detection in as wide a variety of atmospheres as possible? A Centauri Dreams regular, Alex addresses the question by looking at new work on chemical disequilibrium and its relation to biosignature detection. The author (with Brian McConnell) of A Design for a Reusable Water-Based Spacecraft Known as the Spacecoach (Springer, 2016), Alex is a lecturer in biology at the University of California. Just how close are we to an unambiguous biosignature detection, and on what kind of world will we find it?
by Alex Tolley
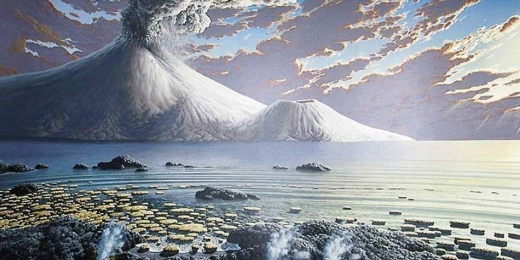
Image: Archaean or early Proterozoic Earth showing stromatolites in the foreground. Credit: Peter Sawyer / Smithsonian Institution.
The Kepler space telescope has established that exoplanets are abundant in our galaxy and that many stars have planets in their habitable zones (defined as having temperatures that potentially allow surface water). This has reinvigorated the quest to answer the age-old question “Are We Alone?”. While SETI attempts to answer that question by detecting intelligent signals, the Drake equation suggests that the emergence of intelligence is a subset of the planets where life has emerged. When we envisage such living worlds, the image that is often evoked is of a verdant paradise, with abundant plant life clothing the land and emitting oxygen to support respiring animals, much like our pre-space age visions of Venus.
Naturally, much of the search for biosignatures has focused on oxygen (O2), whose production on Earth is now primarily produced by photosynthesis. Unfortunately, O2 can also be produced abiotically via photolysis of water, and therefore alone is not a conclusive biosignature. What is needed is a mixture of gases in disequilibrium that can only be maintained by biotic and not abiotic processes. Abiotic processes, unless continually sustained, will tend towards equilibrium. For example, on Earth, if life completely disappeared today, our nitrogen-oxygen dominated atmosphere would reach equilibrium with the oxygen bound as nitrate in the ocean.
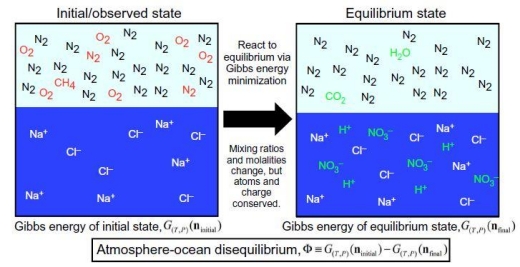
Image: Schematic of methodology for calculating atmosphere-ocean disequilibrium. We quantify the disequilibrium of the atmosphere-ocean system by calculating the difference in Gibbs energy between the initial and final states. The species in this particular example show the important reactions to produce equilibrium for the Phanerozoic atmosphere-ocean system, namely, the reaction of N2, O2, and liquid water to form nitric acid, and methane oxidation to CO2 and H2O. Red species denote gases that change when reacted to equilibrium, whereas green species are created by equilibration. Details of aqueous carbonate system speciation are not shown. Credit: Krissansen-Totton et al. (citation below).
Another issue with looking for O2 is that it assumes a terrestrial biology. Other biologies may be different. However environments with large, sustained, chemical disequilibrium are more likely to be a product of biology.
A new paper digs into the issue. The work of Joshua Krissansen-Totton (University of Washington, Seattle), Stephanie Olson (UC-Riverside) and David C. Catling (UW-Seattle), the paper tackles a question the authors have addressed in an earlier paper:
“Chemical disequilibrium as a biosignature is appealing because unlike searching for biogenic gases specific to particular metabolisms, the chemical disequilibrium approach makes no assumptions about the underlying biochemistry. Instead, it is a generalized life-detection metric that rests only on the assumption that distinct metabolisms in a biosphere will produce waste gases that, with sufficient fluxes, will alter atmospheric composition and result in disequilibrium.”
This approach also opens up the possibility of detecting many more life-bearing worlds as the Earth’s highly oxygenated atmosphere has only been in this state for about 12% of the Earth’s existence.
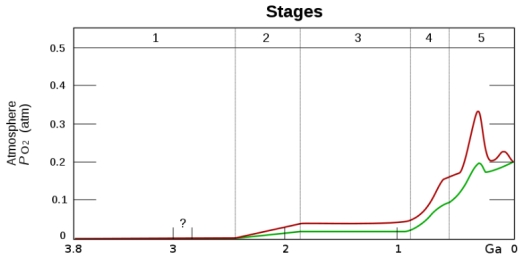
Image: Heinrich D. Holland derivative work: Loudubewe (talk) – Oxygenation-atm.svg, CC BY-SA 3.0,
https://commons.wikimedia.org/w/index.php?curid=12776502
With the absence of high partial pressures of O2 before the Pre-Cambrian, are there biogenic chemical disequilibrium conditions that can be discerned from the state of primordial atmospheres subject to purely abiotic equilibrium?
The new Krissansen-Totton et al? paper attempts to do that for the Archaean (4 – 2.5 gya) and Proterozoic (2.5 – 0.54) eons. Their approach is to calculate the Gibbs Free Energy (G), a metric of disequilibrium, for gases in an atmosphere-oceanic environment. The authors use a range of gas mixtures from the geologic record and determine the disequilibrium they represent using calculations of G for the observed versus the expected equilibrium concentrations of chemical species.
The authors note that almost all the G is in our ocean compartment from the nitrogen (N2)-O2 not reaching equilibrium as ionic nitrate. A small, but very important disequilibrium between methane (CH4) and O2 in the atmosphere is also considered a biosignature.
Using their approach, the authors look at the disequilibria in the atmosphere-ocean model in the earlier Archaean and Proterozoic eons. The geologic and model evidence suggests that the atmosphere was largely N2 and carbon dioxide (CO2), with a low concentration of O2 (2% or less partial pressure) in the Proterozoic.
In the Proterozoic, as today, the major disequilibrium is due to the lack of nitrate in the oceans and therefore the higher concentrations of O2 in the atmosphere. Similarly, an excess concentration of CH4 that should quickly oxidize to CO2 at equilibrium. In the Archaean, prior to the increase in O2 from photosynthesis, the N2, CO2, CH4 and liquid H2O equilibrium should consume the CH4 and increase the concentration of ammonium ions (NH4+ ) and bicarbonate (HCO3-) in the ocean. The persistence of CH4 in both eons is primarily driven by methanogen bacteria.
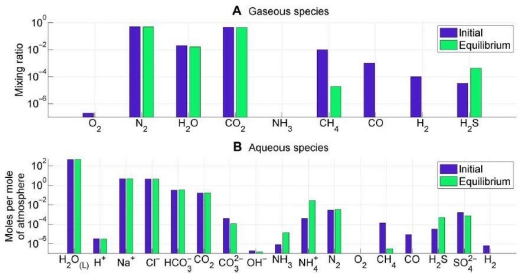
Image: Atmosphere-ocean disequilibrium in in the Archean. Blue bars denote assumed initial abundances from the literature, and green bars denote equilibrium abundances calculated using Gibbs free energy minimization. Subplots separate (A) atmospheric species and (B) ocean species. The most important contribution to Archean disequilibrium is the coexistence of atmospheric CH4, N2, CO2, and liquid water. These four species are lessened in abundance by reaction to equilibrium to form aqueous HCO3 and NH4. Oxidation of CO and H2 also contributes to the overall Gibbs energy change. Credit: Krissansen-Totton et al.
Therefore a biosignature for such an anoxic world in a stage similar to our Archaean era, would be to observe an ocean coupled with N2, CO2 and CH4 in the atmosphere. There is however an argument that might make this biosignature ambiguous.
CH4 and carbon monoxide (CO) might be present due to impacts of bolides (Kasting). Similarly, under certain conditions, it is possible that the mantle might be able to outgas CH4. In both cases, CO would be present and indicative of an abiogenic process. On Earth, CO is consumed as a substrate by bacteria, so its presence should be absent on a living world, even should such outgassing or impacts occur. The issue of CH4 outgassing, at least on earth, is countered by the known rates of outgassing compared to the concentration of CH4 in the atmosphere and ocean. The argument is primarily about rates of CH4 production between abiotic and biotic processes. Supporting Kasting, the authors conclude that on Earth, abiotic rates of production of CH4 fall far short of the observed levels.
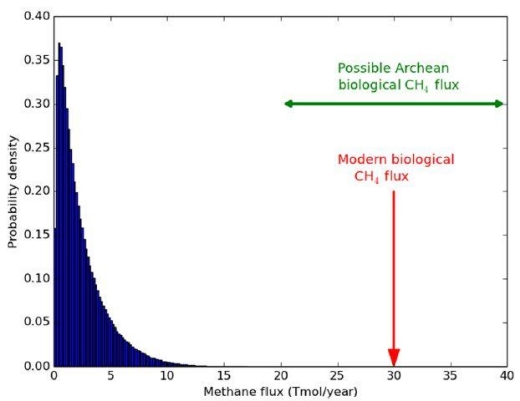
Image: Probability distribution for maximum abiotic methane production from serpentinization on Earth-like planets. This distribution was generated by sampling generous ranges for crustal production rates, FeO wt %, maximum fractional conversion of FeO to H2, and maximum fractional conversion of H2 to CH4, and then calculating the resultant methane flux 1 million times (see the main text). The modern biological flux (58) and plausible biological Archean flux (59) far exceed the maximum possible abiotic flux. These results support the hypothesis that the co-detection of abundant CH4 and CO2 on a habitable exoplanet is a plausible biosignature. Credit: Krissansen-Totton et al.
The authors conclude that their biosignature should also exclude the presence of CO to confirm the observed gases as a biosignature:
“The CH4-N2-CO2-H2O disequilibrium is thus a potentially detectable biosignature for Earth-like exoplanets with anoxic atmospheres and microbial biospheres. The simultaneous detection of abundant CH4 and CO2 (and the absence of CO) on an ostensibly habitable exoplanet would be strongly suggestive of biology.”
Given these gases in the presence of an ocean, can we use them to detect life on exoplanets?
Astronomers have been able to detect CO2, H2O, CH4 and CO in the atmosphere of HD 189733b, which is not Earthlike, but rather a hot Jupiter with a temperature of 1700F, far too hot for life. So far these gases have not been detectable on rocky worlds. Some new ground-based telescopes and the upcoming James Webb Space Telescope should have the capability of detecting these gases using transmission spectroscopy as these exoplanets transit their star.
It is important to note that the presence of an ocean is necessary to create high values of G. The Earth’s atmosphere alone has quite a low G, even compared to Mars. It is the presence of an ocean that results in G orders of magnitude larger than that from the atmosphere alone. Such an ocean is likely to be first detected by glints or the change in color of the planet as it rotates exposing different fractions of land and ocean.
An interesting observation of this approach is that a waterworld or ocean exoplanet might not show these biosignatures as the lack of weathering blocks the geologic carbon cycle and may preclude life’s emergence or long term survival. This theory might now be testable using spectroscopy and calculations for G.
This approach to biosignatures is applicable to our own solar system. As mentioned, Mars’ current G is greater than Earth’s atmosphere G. This is due to the photochemical disequilibrium of CO and O2. The detection of CH4 in Mars’ atmosphere, although at very low levels, would add to their calculation of Mars’ atmospheric G. In future, if the size of Mars’ early ocean can be inferred and gases in rocks extracted, the evidence for paleo life might be inferred. Fossil evidence of life would then confirm the approach.
Similarly, the composition of the plumes from Europa and Enceladus should also allow calculation of G for these icy moons and help to infer whether their subsurface oceans are abiotic or support life.
Within a decade, we may have convincing evidence of extraterrestrial life. If any of those worlds are not too distant, the possibility of studying that life directly in the future will be exciting.
The paper is Krissansen-Totton? et al., “Disequilibrium biosignatures over Earth history and implications for detecting exoplanet life,?” (2018)? Science Advances 4 ? (abstract? / ?full tex?t).
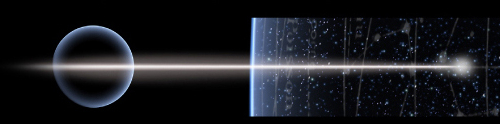
by Paul Gilster | Feb 22, 2018 | Exoplanetary Science |
Between Kepler and the ensuing K2 mission, we’ve had quite a haul of exoplanets. Kepler data have been used to confirm 2341 exoplanets, with NASA declaring 30 of these as being less than twice Earth-size and in the habitable zone. K2 has landed 307 confirmed worlds of its own. K2 offers a different viewing strategy than Kepler’s fixed view of over 150,000 stars. While the transit method is still at work, K2 pursues a series of observing campaigns, its fields of view distributed around the ecliptic plane, and with photometric precision approaching the original.
Why the relationship with the ecliptic? Remember that what turned Kepler into K2 was the failure of two reaction wheels, the second failing less than a year after the first. Working in the ecliptic plane minimizes the torque produced by solar wind pressure, thus minimizing pointing drift and allowing the spacecraft to be controlled by its thrusters and remaining two reaction wheels. Each K2 campaign is limited to about 80 days because of sun angle constraints.
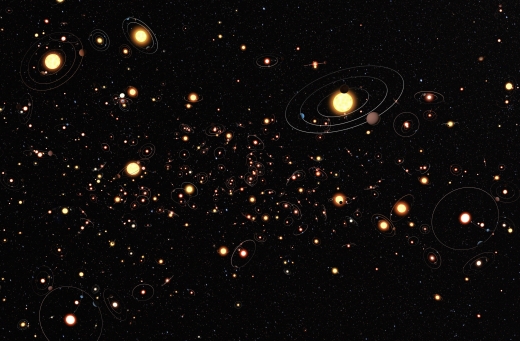
Image: After detecting the first exoplanets in the 1990s, astronomers have learned that planets around other stars are the rule rather than the exception. There are likely hundreds of billions of exoplanets in the Milky Way alone. Credit: ESA/Hubble/ESO/M. Kornmesser.
More K2 planets have now turned up in an international study led by Andrew Mayo (National Space Institute, Technical University of Denmark). The research, underway since the first release of K2 data in 2014, uncovered 275 planet candidates, of which 149 were validated. 56 of the latter had not previously been detected, while 39 had already been identified as candidates, and 53 had already been validated, with one previously classed as a false positive.
Overall, the work increases the validated K2 planet sample by almost 50 percent, while increasing the K2 candidate sample by 20 percent. What stands out here is not so much the trove of new planets but the validation techniques brought to bear, which were applied to a large sample as part of a framework developed to increase validation speed. From the paper:
This research will also be useful even after the end of the K2 mission. The upcoming TESS mission (Ricker et al. 2015) is expected to yield more than 1500 total exoplanet discoveries, but it is also estimated that TESS will detect over 1000 false positive signals (Sullivan et al. 2015). Even so, one (out of three) of the level one baseline science requirements for TESS is to measure the masses of 50 planets with Rp < 4 R?. Therefore, there will need to be an extensive follow-up program to the primary photometric observations conducted by the spacecraft, including careful statistical validation to aid in the selection of follow-up targets. The work presented here will be extremely useful in that follow-up program, since only modest adjustments will allow for the validation of planet candidate systems identified by TESS rather than K2.
The work involves not only analysis of the K2 light curves but also follow-up spectroscopy and high contrast imaging involving ground-based observation of candidate host stars. The processes of data reduction, candidate identification, and statistical validation described here using a statistical validation tool called vespa clearly have application well beyond K2.
The paper is Mayo et al., “275 Candidates and 149 Validated Planets Orbiting Bright Stars in K2 Campaigns 0-10,” accepted at the Astronomical Journal (preprint).
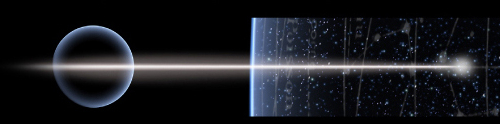
by Paul Gilster | Feb 21, 2018 | Astrobiology and SETI |
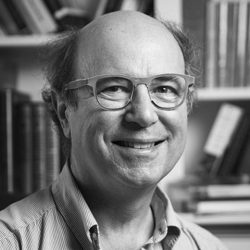
Frank Wilczek has used the neologism ‘quintelligence’ to refer to the kind of sentience that might grow out of artificial intelligence and neural networks using genetic algorithms. I seem to remember running across Wilczek’s term in one of Paul Davies books, though I can’t remember which. In any case, Davies has speculated himself about what such intelligences might look like, located in interstellar space and exploiting ultracool temperatures.
A SETI target? If so, how would we spot such a civilization?
Wilczek is someone I listen to carefully. Now at MIT, he’s a mathematician and theoretical physicist who was awarded the Nobel Prize in Physics in 2004, along with David Gross and David Politzer, for work on the strong interaction. He’s also the author of several books explicating modern physics to lay readers. I’ve read his The Lightness of Being: Mass, Ether, and the Unification of Forces (Basic Books, 2008) and found it densely packed but rewarding. I haven’t yet tackled 2015’s A Beautiful Question: Finding Nature’s Deep Design.
Perhaps you saw Wilczek’s recent piece in The Wall Street Journal, sent my way by Michael Michaud. Here we find the scientist going at the Fermi question that we have tackled so many times in these pages, always coming back to the issue that we have a sample of one when it comes to life in the universe, much less technological society, and our sample is right here on Earth. For the record, Wilczek doesn’t buy the idea that life is unusual; in fact, he states not only that he thinks life is common, but also makes the case for advanced civilizations:
Generalized intelligence, that produces technology, took a lot longer to develop, however, and the road from amoebas to hominids is littered with evolutionary accidents. So maybe we’re our galaxy’s only example. Maybe. But since evolution has supported many wild and sophisticated experiments, and because the experiment of intelligence brings spectacular adaptive success, I suspect the opposite. Plenty of older technological civilizations are out there.
Civilizations may, of course, develop and then, in Wilczek’s phrase, ‘flame out,’ just as Edward Gibbon would describe the fall of Rome as “the natural and inevitable effect of immoderate greatness. . . . The stupendous fabric yielded to the pressure of its own weight.” We can pile evidence onto that one, from the British and Spanish empires to the decline of numerous societies like the Aztec and the Mayan. Catastrophe is always a possible human outcome.
But is it an outcome for non-human technological societies? Wilczek doubts that, preferring to hark back to the idea with which we opened. The most advanced quantum computation — quintelligence — he believes, works best where it is cold and dark. And a civilization based on what we would today call artificial intelligence may be one that basically wants to be left alone.
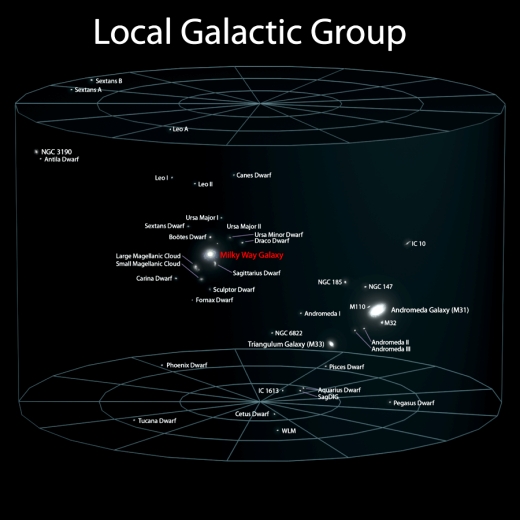
Image: The Local Group of galaxies. Is the most likely place for advanced civilization to be found in the immensities between stars and galaxies? Credit: Andrew Z. Colvin.
All this is, like all Fermi question talk, no more than speculation, but it’s interesting speculation, for Wilczek goes on to discuss the notion that one outcome for a hyper-advanced civilization may be to embrace the small. After all, the speed of light is a limit to communications, and effective computation involves communications that are affected by that limit. The implication: Fast AI thinking works best when it occurs in relatively small spaces. Thus:
Consider a computer operating at a speed of 10 gigahertz, which is not far from what you can buy today. In the time between its computational steps, light can travel just over an inch. Accordingly, powerful thinking entities that obey the laws of physics, and which need to exchange up-to-date information, can’t be spaced much farther apart than that. Thinkers at the vanguard of a hyper-advanced technology, striving to be both quick-witted and coherent, would keep that technology small.
A civilization based, then, on information processing would achieve its highest gains by going small in search of the highest levels of speed and integration. We’re now back out in the interstellar wastelands, which may in this scenario actually contain advanced and utterly inconspicuous intelligences. As I mentioned earlier, it’s hard to see how SETI finds these.
Unstated in Wilczek’s article is a different issue. Let’s concede the possibility of all but invisible hyper-intelligence elsewhere in the cosmos. We don’t know how long it would take to develop such a civilization, moving presumably out of its initial biological state into the realm of computation and AI. Surely along the way, there would still be societies in biological form leaving detectable traces of themselves. Or should we assume that the Singularity really is near enough that even a culture like ours may be succeeded by AI in the cosmic blink of an eye?
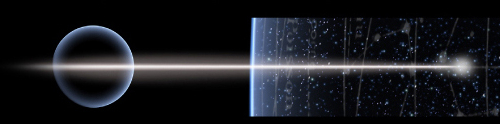