by Paul Gilster | Oct 31, 2011 | Exoplanetary Science |
Long-time Centauri Dreams readers will be familiar with the work of Manoj Joshi and Robert Haberle. Back in the 1990s when both were at NASA Ames (Joshi is now at the University of Reading), the scientists went to work on the question of whether planets around red dwarf stars could be habitable, given the problem of close orbits and tidal lock. Simulating the atmosphere of such a planet, they found even a thin atmosphere would circulate globally, moving enough heat to prevent the air on the darkside from freezing out. The prospect of a planet with oceans and a climate mild enough to support life began to look more promising.
Joshi and Haberle have a new paper out that looks once again at planets around red dwarfs, this time extending the possible habitable zone to a greater distance from the star. M-class red dwarfs are smaller and cooler than G-class stars like the Sun, and emit a much larger fraction of their radiation at longer wavelengths where the reflectivity of ice and snow are lower. The effect is striking, as the paper notes:
The values for snow and ice for a planetary surface orbiting the Sun are 0.8 and 0.5 respectively, which are broadly consistent with the values that are used in climate models. Fresh snow and ice albedos on a planet receiving black body radiation from an object at 3300K are 0.6 and 0.3 respectively, which are significant reductions from the “solar” values.
The black body radiation is an idealised representation of an M-dwarf that is approximately 40% as massive as the Sun. The authors then go on to calculate the albedos for snow and ice on hypothetical planetary surfaces around the stars Gliese 436 and GJ 1214 and find them even lower. What this means is that more of the long-wave radiation emitted by an M-dwarf will be absorbed rather than reflected by an icy surface. The effect is to widen the habitable zone of a planet around this kind of star outwards by anywhere from 10 to 30 percent. What might have seemed a frigid world now looks more hospitable.
Allowing a habitable zone (defined here in terms of liquid water at the surface) to exist farther out from the parent star is significant, although there are no changes at the other end of the HZ:
The effect considered here should not move the inner edge of the habitable zone, usually considered as the locus of orbits where loss rates of water become significant to dry a planet on geological timescales (Kasting et al 1993), away from the parent M-star. This is because when a planet is at the inner edge of the habitable zone, surface temperatures should be high enough to ensure that ice cover is small. For a tidally locked planet this implies that ice is confined to the dark side that perpetually faces away from the parent star: such ice receives no stellar radiation, rendering albedo effects unimportant.
But planets with significant amounts of snow and ice will have higher surface temperatures and the outer edge of the habitable zone is extended. It’s an interesting thought because of the sheer ubiquity of red dwarfs — some estimates of their prevalence run as high as 80 percent of main-sequence stars, so seeing them as astrobiologically friendly would revise our estimates for extraterrestrial life. Just how close the nearest life-bearing planet might be affects our planet hunt astronomically as we choose targets and has significance for any future interstellar probes.
Other issues remain and are under active investigation, from the problem of flares to climate models involving the effects of clouds and water vapor. A good place to get an overview is Tarter et al., “A Reappraisal of The Habitability of Planets around M Dwarf Stars,” Astrobiology 7, pp 30-65 (2007). The Joshi and Haberle paper is “Suppression of the water ice and snow albedo feedback on planets orbiting red dwarf stars and the subsequent widening of the habitable zone,” accepted by Astrobiology (preprint).
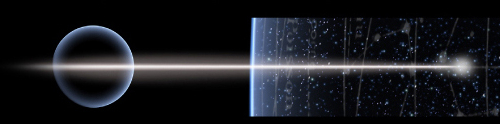
by Paul Gilster | Oct 28, 2011 | Outer Solar System |
?Sometimes our spacecraft take us past an asteroid, and other times the asteroid comes to us. Asteroid 2005 YU55 pays Earth a visit soon, closing to a bit less than the distance of the Moon on November 8. The Deep Space Network dish at Goldstone (CA) and the Arecibo facility in Puerto Rico will track the object, with images from Goldstone expected to achieve resolutions as fine as 2 meters per pixel, which should give us a wealth of information about the asteroid’s surface features. About the size of an aircraft carrier, 2005 YU55 was also observed by Arecibo in 2010, when it was found to be roughly spherical in shape with a rotation period of 18 hours.
You’ll also recall asteroid 21 Lutetia, which we covered here when the European Space Agency’s Rosetta probe flew past it in July of 2010. The imagery from that encounter showed a cracked and battered surface, but new analysis now indicates that the asteroid may once have had a hot metallic core. Lutetia may, in other words, be a remnant of an ancient period of planetary formation.
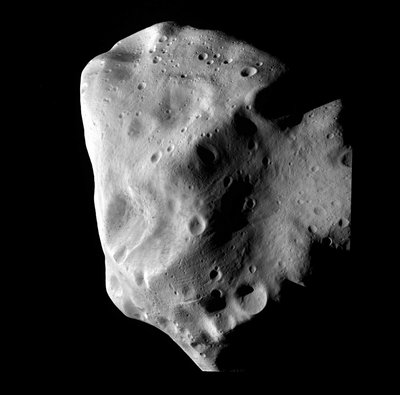
This is interesting stuff because most objects in the main belt between Mars and Jupiter are thought to be relatively light masses of rock and metal that have battered each other for billions of years. Few would have melted to form metallic cores, so the thinking goes, and most would be little more than loosely bound piles of debris. But Holger Sierks (Max-Planck Institute for Solar System Research) and the Rosetta team find that Lutetia is actually quite dense. They based their work on calculations of the asteroid’s volume and mass, drawing on models derived from the Rosetta images and the effect of Lutetia’s gravity on the spacecraft.
Image: A year after the flyby, the analysis shows that Lutetia is a primordial asteroid, with a surface covered in craters, pulverised rocks and landslides. It may also have tried to form an iron core billions of years ago. Credit: ESA 2010 MPS for OSIRIS Team MPS/UPD/LAM/IAA/RSSD/INTA/UPM/DASP/IDA.
The Sierks paper on this work puts the matter succinctly:
[Lutetia’s] geologically complex surface, ancient surface age, and high density suggest that Lutetia is most likely a primordial planetesimal. This contrasts with smaller asteroids visited by previous spacecraft, which are probably shattered bodies, fragments of larger parents, or reaccumulated rubble piles.
Look at the surface of Lutetia and you see huge fractures that suggest a porous object that does not square with the density calculations. But Benjamin Weiss (MIT) suggests that melted material beneath the fractured crust — a dense metallic core — would account for the discrepancy. And instead of the idea of asteroids as primordial unmelted objects, we begin to see a boundary line, with the possibility of larger asteroids being partially differentiated, possessing a melted interior overlain by outer layers. Here’s Weiss on the subject:
“The planets don’t retain a record of these early differentiation processes. So this asteroid may be a relic of the first events of melting in a body.”
That would make asteroids like Lutetia unusual but not unique — there may be many objects with unmmelted surfaces but differentiated interiors. The challenge will be to get to an asteroid to return samples that could provide more evidence for this explanation. The smallest asteroids are unlikely to retain a molten interior, but larger bodies in the asteroid belt may prove more interesting than we had realized.
At the time of the 2010 encounter, Lutetia was the largest asteroid ever to have been visited by a spacecraft. Vesta has now eclipsed that record, and the recent findings will make Dawn’s missions at Vesta and Ceres all the more interesting. An asteroid sample return mission — Weiss is part of the NASA team — is slated for a 2016 launch, though not to Lutetia. It’s interesting to note here that earlier work by Weiss on the Allende meteorite showed that samples were strongly magnetized, leading to the theory that the meteorite came from an asteroid with a melted metallic core. All of this may be showing us planetary development in its earliest stages.
The papers are Sierks et al., “Images of Asteroid 21 Lutetia: A Remnant Planetesimal from the Early Solar System,” Science Vol. 334 no. 6055, pp. 487-490 (28 October 2011), abstract available. See also Pätzold et al., “Asteroid 21 Lutetia: Low Mass, High Density,” Science Vol. 334 no. 6055, pp. 491-492 (28 October 2011) — abstract — and Coradini et al., “The Surface Composition and Temperature of Asteroid 21 Lutetia As Observed by Rosetta/VIRTIS,” Science Vol. 334 no. 6055, pp. 492-494 (28 October 2011), abstract available. Weiss’ paper on differentiation is Weiss et al., “Possible evidence for partial differentiation of asteroid Lutetia from Rosetta,” Planetary and Space Science, in press, available online 8 October 2011 (abstract).
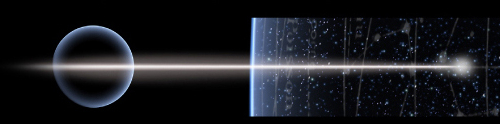
by Paul Gilster | Oct 27, 2011 | Outer Solar System |
Back when I was writing my Centauri Dreams book in 2004, I remember talking to JPL’s James Lesh about various aspects of communicating with distant spacecraft. Lesh had written an interesting paper on how we might communicate with an Alpha Centauri probe, and we went on to discuss laser communications in the context of today’s Deep Space Network. What stuck in my mind about that conversation was how much we can learn when one thing moves in front of another. Lesh pointed out how useful it is to examine a radio signal when a spacecraft moves behind a planet, studying the attenuation of the signal and learning more about the planet itself.
When a celestial object moves in front of a distant star, we also get useful results, as has recently happened in our studies of the dwarf planet Eris. You’ll recall that it was Eris that kicked off a new round of controversy about Pluto, for the early estimates were that the diameter of Eris (then thought to be 3000 kilometers) was actually 25 percent larger than Pluto’s. The possibility of numerous such objects in the Kuiper Belt caused us to reassess the definition of the word ‘planet,’ leading to the creation of ‘dwarf planet’ as the operative term for both Eris and Pluto.
But in November of last year, Eris occulted a distant star, an event monitored at two sites in Chile, including the TRAPPIST (TRAnsiting Planets and PlanetesImals Small Telescope) at La Silla. We learn from this that Eris is close to spherical, assuming there are no large mountains on the object, and that Pluto and Eris are more or less the same size, with the new diameter calculated for Eris coming in at 2326 kilometers (Pluto’s is between 2300 and 2400 kilometers). It’s interesting that Pluto’s diameter is actually harder to measure because even with the help of past occultations, the presence of an atmosphere makes the measurement less precise.
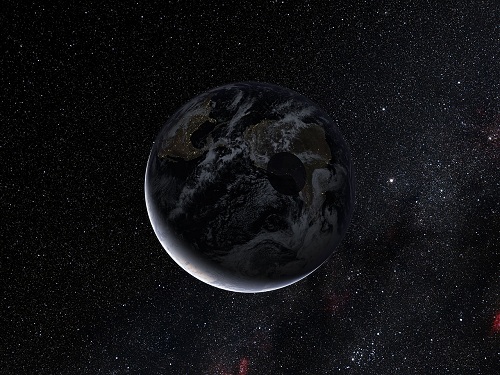
Image: This artist’s impression shows the shadow of the dwarf planet Eris as it was crossing the Earth during the occultation in November of 2010. The regions along the path saw a faint star briefly disappear as its light was blocked by Eris. Studies of where the event was seen, and for how long, have allowed astronomers to measure the size of Eris accurately for the first time. Surprisingly, they find it to be almost exactly the same size as Pluto and learn that it has a very reflective surface. Credit: ESO/L. Calçada.
Eris turns out to be reflective indeed, with an albedo of 0.96 (reflecting some 96 percent of the light that falls on it). This ESO news release notes that this albedo makes the dwarf planet brighter than fresh snow on Earth, flagging it as one of the most reflective objects in the Solar System, in company with Saturn’s moon Enceladus. Moreover, studies of Eris’ moon Dysnomia help us arrive at a mass estimate for Eris, which turns out to be 27 percent heavier than Pluto, with a density estimated at 2.52 grams per cubic centimeter. Emmanuel Jehin, one of the researchers involved in the occultation study, says this about the finding:
“This density means that Eris is probably a large rocky body covered in a relatively thin mantle of ice. This layer of ice could result from the dwarf planet’s nitrogen or methane atmosphere condensing as frost onto its surface as it moves away from the Sun in its elongated orbit and into an increasingly cold environment.”
Estimates suggest a surface temperature of -238 Celsius on the day side of Eris, but when the object moves back toward perihelion in its orbit (37.8 AU), the ice Jehin is talking about may well turn back into gas. Eris is currently at 96.6 AU, near aphelion. Telescopes at 26 different locations around the globe were used to observe the occultation, but the dwarf planet’s shadow was only detected at the two sites in Chile, where a sudden drop in brightness was apparent. But even with small telescopes, the value of one celestial object moving in front of another is again demonstrated, helping us learn more about a dwarf planet on the edge of the Solar System.
The paper is Sicardy et al., “A Pluto-like radius and a high albedo for the dwarf planet Eris from an occultation,” Nature 478, 493-496 (27 October 2011). Abstract available.
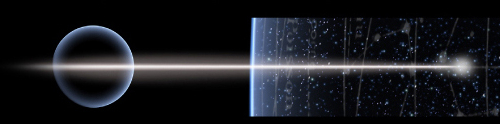
by Paul Gilster | Oct 26, 2011 | Exoplanetary Science |
Imaging an Earth-like planet in the habitable zone may happen some time in the next decade if the James Webb Space Telescope can make its way through its budgetary hurdles and achieve a 2018 liftoff. But the word ‘imaging’ is a bit deceptive when you consider that we won’t be getting anything remotely like the view of a planet in our own Solar System through Webb’s instruments. No small disc with discernible features, in other words, but a single dot useful not because of what we can pick out visually, but because we can use its light to take a spectrum. And if we’re really lucky, we’ll find a dot that’s blue and a spectrum that shows the signature of a living world.
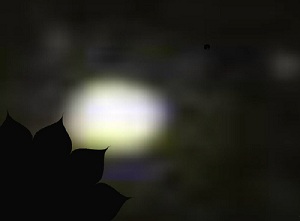
The JWST works at infrared wavelengths (covering a range from 0.6 to 28 micrometers), which is why shielding it from heat is so important, and why the design is marked by a large sunshield. These wavelengths should allow the instrument to study stars and galaxies from the early universe, but the addition of a starshade to the mission concept would enhance the exoplanet part of the conversation. In a recent Astrobiology Magazine article, Leslie Mullen talked to Matt Mountain and John Grunsfeld (Space Telescope Science Institute) about what a starshade might do (thanks to Erik Anderson for the tip). Think of a disc tens of meters wide with petal-like extensions placed between telescope and star. Mountain likened the starshade to putting your thumb in front of the Sun to create shadow:
[The starshade is] very carefully shaped, so you don’t get the sort of flaring that you normally get when you use a perfect sphere, where you get all these rings and refractions. These petals are designed to create a very smooth, very deep shadow. You basically slide in and out of the shadow, and then you can actually see the planet next to the star. The star is in the shadow, and the planet peeks around the shadow.
Image: A starshade would obscure the light of a star to allow its planets to become visible. Credit: Webster Cash/University of Colorado, Boulder.
The starshade would be placed 160,000 kilometers away from the JWST, which will orbit at the L2 Lagrangian point. And as Grunsfeld points out, that single dot yields other information:
…if you had enough time, and there were seasons, with ice covering and then going away, you could study it and be able to tell the difference between winter and summer on the planet, or vegetation, in principle. Just from unresolved single pixels, because of the color changes.
Given our inability to get a finalized Terrestrial Planet Finder design into the mix, the JWST thus becomes a major exoplanet asset, though a mission of daunting complexity, and one whose orbit would make any necessary on-site repair problematic, to say the least. Unlike Kepler, this is an instrument that can sample stars that are relatively near to the Earth. Most of the 150,000 stars in the Kepler field are further away, chosen because the goal of Kepler is to study a large number so as to achieve a statistical analysis of the incidence of the various kinds of planets around them.
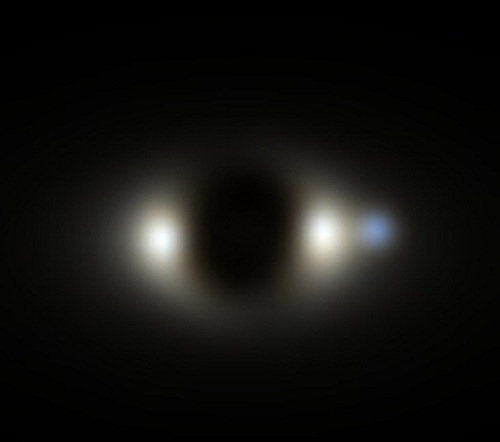
Image: What a starshade might see, based on studies for a starshade concept called New Worlds Observer, one we’ve discussed many times on Centauri Dreams. The image shows an Earth-like planet at a distance of 30 light years. The white ring is dust in the stellar system, reflecting starlight under an angle of 30°. The central black disk is the shadow cast by the starshade. The Earth-like planet is the object with the familiar blue hue. Credit: Phil Oakley, Webster Cash (University of Colorado, Boulder).
Adding a starshade to the JWST mission profile adds to both cost and complexity, but the starshade would extend the telescope’s ability to see exoplanets. On its own, JWST can study a transiting world, but Mountain figures such transits might show up in no more than 5 to 7 percent of stars. The ability to take a planetary spectrum independent of the planet’s orientation around the star is what the starshade offers. We now wait to see whether JWST itself has a future. Its ballooning costs have threatened the project in Congress, but NASA administrator Charlie Bolden sees the telescope as one of the agency’s top priorities. With $3.5 billion already spent and ¾ of the construction and testing complete, Centauri Dreams thinks this mission will fly, but not without the kind of budgetary near-miss that is the nemesis of complex science everywhere. The loss of the Space Interferometry Mission will always be a reminder of what can still happen.
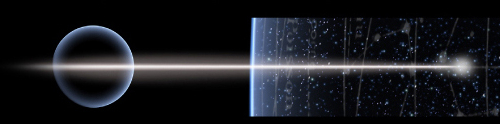
by Paul Gilster | Oct 25, 2011 | Sail Concepts |
Back in August I mentioned NASA’s solar sail plans beyond NanoSail-D in the context of a larger survey of sail designs and experimentation. It’s great to see multiple sail projects in motion, and before I return to NASA I should mention not only the Planetary Society’s ongoing sail effort but the CubeSat sail being built by a consortium from the University of Surrey and aerospace firm Astrium, an aerospace subsidiary of the European Aeronautic Defence and Space Company (EADS). Then there’s the German space agency DLR and its Gossamer sails, experimental designs being worked on with the European Space Agency. Surely energized by the success of the Japanese IKAROS sail, work on this fledgeling space technology is beginning to ramp up.
NASA’s next step in sail design builds upon the earlier work the agency performed with aerospace contractor L’Garde Inc., of Tustin, California, which deployed and tested a 20m X 20m sail at the agency’s Plumbrook facility in Ohio. The plan is to build a solar sail demonstration mission that will create a sail quadruple the size of the Plumbrook sail and conduct operations in space to demonstrate attitude control as well as passive stability and trim adjustment using beam-tip vanes. The demonstrator mission will also allow the craft to execute basic navigation operations.
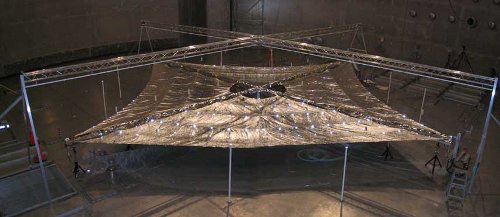
Image: A four-quadrant solar sail system sits fully deployed in a 100-foot-diameter vacuum chamber at NASA’s Glenn Research Center Plum Brook Station in Sandusky, Ohio. NASA’s solar sail propulsion team at the Marshall Space Flight Center in Huntsville, Ala., and its industry partner, L’Garde, Inc., of Tustin, Calif., successfully deployed the solar sail system during testing at the Plum Brook facility in early July 2004. The tests included temperatures as cold as minus 112 degrees Fahrenheit to simulate conditions of open space. Credit: NASA/L’Garde, Inc.
Technology demonstrator missions like this one provide near-term spacecraft that can show the feasibility of new technologies, and that means flight hardware tested in space. The plan is for the sail mission to piggyback with other payloads aboard a commercially available launch vehicle, with launch scheduled for 2015 or 2016, and a one to two year period of spacecraft operations and analysis. NASA talks about future solar sail capabilities in the realm of satellite deorbiting (essentially using the sail as part of a satellite payload for this purpose) and station-keeping, allowing ‘pole-sitter’ sails, for example, for geosynchronous high-latitude operations.
The final purpose, as noted in this NASA news release, is to develop sails for deep space propulsion, but we’ll need to build up a sail capability much closer to home before we can think of committing serious payloads for these purposes. We’ll doubtless see attempts at something like GeoStorm, which would place solar storm warning satellites at positions between the Earth and the Sun to increase our warning time for solar flares. The demonstrator sail is a precursor to all these applications, and NASA’s work with L’Garde and the National Oceanic and Atmospheric Administration should be seen as part of firming up sail techniques as we aim for bigger missions.
Another part of that process would be to move into space-based experimentation on microwave beaming. Back when the Planetary Society was planning for the ill-fated Cosmos 1 sail (lost evidently without achieving orbit in a booster accident), the plans included an attempt to measure the effect of microwaves on the sail using the Goldstone dish. It would be heartening to see this kind of thinking continued in the next round of missions. My own take is that beamed sails offer huge advantages for deep space, including not just the fact that the spacecraft does not carry fuel onboard but that the physics of microwave beaming to a sail are comparatively well understood. More on beamed sail concepts soon as we look at some of Jim Benford’s thoughts on the idea.
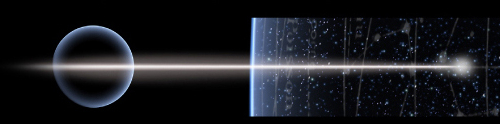