by Paul Gilster | Feb 28, 2014 | Exoplanetary Science |
The Kepler announcements yesterday were greatly cheering to those of us fascinated with the sheer process of doing exoplanetology. The ‘verification by multiplicity’ technique propelled the statistical analysis that resulted in 715 newly verified worlds, and we have yet to turn it loose on two more years of Kepler data (check Hugh Osborn’s excellent Lost in Transits site for more on the method). For those who focus primarily on habitable worlds, the results seemed a bit more sparse, with just four planets found in the habitable zone. And even where we find such, there are reasons to wonder whether a ‘super-Earth’ could actually sustain life.
Apropos of this question, a team of researchers led by Helmut Lammer (Austrian Academy of Sciences) has just published the results of its modeling of planetary cores, looking at the rate of hydrogen capture and removal for cores between 0.1 and 5 times the mass of the Earth found in the habitable zone of a G-class star. Cores like these inevitably attract hydrogen from the surrounding protoplanetary disk, although some of it will be stripped away by ultraviolet light from the hot young star they orbit. The question is, can enough of this primordial hydrogen envelope be blown off to allow the formation of a more benign secondary atmosphere?
The results are not promising for even relatively small super-Earths. Planetary cores with a mass similar to the Earth capture a hydrogen envelope and can also lose it — the paper suggests that terrestrial planets like Mercury, Venus, Earth and Mars lost their proto-atmospheres because of ultraviolet light. But high-mass cores similar to many of the super-Earth discoveries like Kepler-62e and -62f, wind up with atmospheres much thicker than ours. The habitable zone isn’t enough to guarantee a habitable world, as Lammer notes:
“Our results suggest that worlds like these two super-Earths may have captured the equivalent of between 100 and 1000 times the hydrogen in the Earth’s oceans, but may only lose a few percent of it over their lifetime. With such thick atmospheres, the pressure on the surfaces will be huge, making it almost impossible for life to exist.”
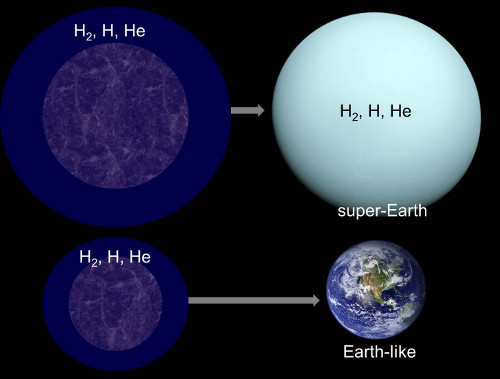
Image: The mass of the initial rocky core determines whether the final planet is potentially habitable. On the top row of the diagram, the core has a mass of more than 1.5 times that of the Earth. The result is that it holds on to a thick atmosphere of hydrogen (H), deuterium (H2) and helium (He). The lower row shows the evolution of a smaller mass core, between 0.5 and 1.5 times the mass of the Earth. It holds on to far less of the lighter gases, making it much more likely to develop an atmosphere suitable for life. Credit: NASA / H. Lammer.
The constraints here get to be pretty tight. Let me quote the paper on this:
Therefore we suggest that ‘rocky’ habitable terrestrial planets, which can lose their nebula-captured hydrogen envelopes and can keep their outgassed or impact delivered secondary atmospheres in habitable zones of G-type stars, have most likely core masses with 1±0.5M? and corresponding radii between 0.8–1.15R?. Depending on nebula conditions, the formation scenarios, and the nebula life time, there may be some planets with masses that are larger than 1.5M? and lost their protoatmospheres, but these objects may represent a minority compared to planets in the Earth-mass domain.
The super-Earths we’re likely to find in habitable zones, according to this work, are going to be uninhabitable places with hydrogen dominated atmospheres. The paper goes on to note that, based on its results, there may well be Earth-size and mass planets that have not been able to shed their captured, nebula-based hydrogen envelope. The upshot: Extreme caution is advised when speculating about how habitable planets evolve, particular when mass and density are still problematic. The variety of outcomes is wide even for small worlds inside the habitable zone.
The paper is Lammer et al., “Origin and Loss of nebula-captured hydrogen envelopes from “sub”- to “super-Earths” in the habitable zone of Sun-like stars,” accepted at Monthly Notices of the Royal Astronomical Society (preprint).
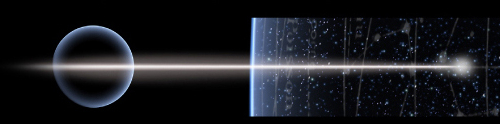
by Paul Gilster | Feb 27, 2014 | Exoplanetary Science |
A planet like Kepler-296f is bound to get a lot of publicity. Orbiting a star half the Sun’s size and only five percent as bright, this world, twice the size of the Earth, appears to orbit in the habitable zone, where liquid water could exist on its surface. We focus so much on the potential of life that the four planets announced yesterday (out of 715 newly verified worlds) inevitably get special treatment. And we learn that Kepler-296f exists in a system with four other planets, orbiting the star every thirty days. What we don’t know is whether we’re dealing with a small Neptune-class world surrounded by a thick hydrogen/helium atmosphere or a water world with a deep ocean.
An interesting world, to be sure, but the real story in yesterday’s announcements from the Kepler team has to do with the ‘verification by multiplicity’ technique used to validate the existence of so many planets in 305 star systems. One of the findings papers titled “Almost All of Kepler’s Multiple Planet Candidates are Planets” (to be published March 10 in The Astrophysical Journal) makes the case that the vast majority of Kepler’s multiple planet candidates are true multi-planet systems, the number of false positives lower for multiples than for single detections. The paper continues (internal citations removed for brevity):
Kepler has found far more multiple candidate systems than would be the case if candidates were randomly distributed among target stars. False positives are expected to be nearly randomly distributed among Kepler targets, whereas true transiting planets could be clustered if planets whose sizes and periods are adequate for transits to be detected often come in multiples, as is the case for planets detected by radial velocity variations, and/or if planetary systems tend to be flat, so geometry leads to higher transit probabilities for other planets if one planet is seen to transit.
The paper gives a blow by blow description of the statistical analysis used here, but for our purposes, Kepler looked at over 150,000 stars and found only a few thousand that showed the characteristic lightcurve of a planetary transit. If this transit-like pattern were random, only a few stars would have shown more than one pattern, but we find hundreds of stars that have multiple transit-like patterns. As Jack Lissauer (NASA Ames) pointed out in yesterday’s teleconference, multiplicity is not random. Instead, it is a technique for opening up what Lissauer calls the ‘planetary verification bottleneck.’ Joining Lissauer in the teleconference were Jason Rowe (SETI Institute), Douglas Hudgins (NASA Astrophysics Division), and Sara Seager (MIT).
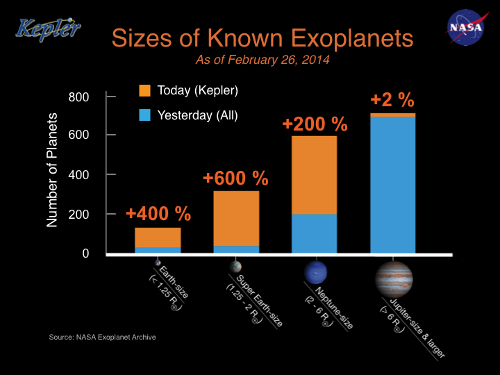
Image: The histogram shows the number of planets by size for all known exoplanets. The blue bars on the histogram represents all the exoplanets known, by size, before the Kepler Planet Bonanza announcement on Feb. 26, 2014. The gold bars on the histogram represent Kepler’s newly-verified planets. Image Credit: NASA Ames/W Stenzel.
Lissauer talks about the method as a way ‘to verify multiple planet candidates in bulk to deliver planets wholesale,’ and it yields 715 worlds of which 94 percent are smaller than Neptune, in the process establishing that multiple planet systems around a single star are common. The number of confirmed exoplanets now nears 1700. Besides Kepler-296f, three other verified worlds are less than 2.5 times the size of Earth and orbiting in their star’s habitable zone.
Overall, these results offer the largest number of validated planets ever to be announced at one time, a number sufficient for the transit technique to overtake radial velocity as the most prolific technique for exoplanet detection. And as Jason Rowe pointed out, these results show us small planets in multiple planet systems that move in circular, flat orbits, a configuration not unlike what we find in our own inner Solar System. “The more we explore,” Rowe added, “the more we find familiar traces of ourselves amongst the stars that remind us of home.”
The paper cited above is Lissauer et al. “Almost All of Kepler’s Multiple Planet Candidates Are Planets,” in press at The Astrophysical Journal (preprint). See also Rowe et al. “Validation of Kepler’s Multiple Planet Candidates. III: Light Curve Analysis & Announcement of Hundreds of New Multi-planet Systems,” also in press at The Astrophysical Journal (preprint). The archived Kepler media teleconference can be accessed here.
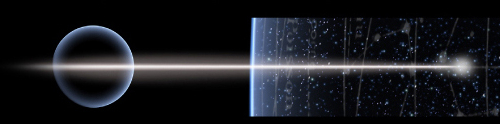
by Paul Gilster | Feb 26, 2014 | Culture and Society |
I’ve had pulsars on the mind the last couple of days after our discussion of PSR 1257+12 and its contribution to exoplanetology. A bit more about pulsars today and the way we look at extreme objects through science fiction. PSR 1257+12 was discovered in 1990 by Aleksander Wolszczan using data from the Arecibo dish, and it was in 1992 that Wolszczan and Dale Frail published a paper outlining their discovery of the first planets ever found outside our Solar System. The two planets were joined by a third in 1994, but evidence for a fourth was later shown to be mistaken. In any case, the three planets confounded many astronomers, who hardly expected the first extrasolar planets to be found orbiting a radiation-spewing neutron star.
Centauri Dreams regular Al Jackson was co-author of a 1992 study of PSR1257+12 that examined orbital resonance in the planets around the pulsar. In a note last night, Al mused “Just think of a K2 civilization setting up a research station on one of those to study a pulsar – maybe someone has written the SF story?” I can’t think of a story specifically targeting a pulsar planet, though if memory serves, Alastair Reynolds deals with a pulsar in one of the early Revelation Space novels. But the notion reminds me of other extreme environment classics like Hal Clement’s Mission of Gravity (1954), which covers the fantastically spinning world Mesklin, where surface gravity gets up close to 700 g at the poles (the complete Mesklin material is available in the collection Heavy Planet: The Classic Mesklin Stories, an Orb title from 2002).
High-energy environments are made to order for hard science fiction. Consider what our best authors might do with this: The pulsar IGR J11014-6103, now the subject of observations by the Chandra X-Ray Observatory, is producing a jet of high-energy particles that this Chandra news release claims is the longest of any object in the Milky Way, a whopping 37 light years. The pulsar is moving away from a supernova remnant in the constellation of Carina at a speed somewhere between 1100 and 2200 kilometers per second, making it one of the fastest moving pulsars yet observed.
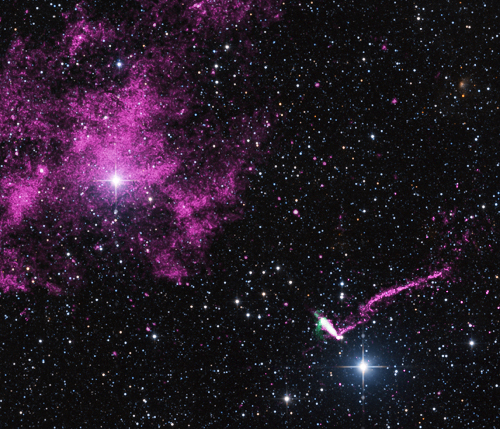
Image: An extraordinary jet trailing behind a runaway pulsar is seen in this composite image that contains data from NASA’s Chandra X-ray Observatory (purple), radio data from the Australia Telescope Compact Array (green), and optical data from the 2MASS survey (red, green, and blue). The pulsar – a spinning neutron star – and its tail are found in the lower right of this image. The tail stretches for 37 light years, making it the longest jet ever seen from an object in the Milky Way galaxy. Credit: X-ray: NASA/CXC/ISDC/L.Pavan et al, Radio: CSIRO/ATNF/ATCA Optical: 2MASS/UMass/IPAC-Caltech/NASA/NSF.
A 37 light-year jet is remarkable enough (nine times the distance to Proxima Centauri!), but we also find that there is a corkscrew pattern in the jet suggesting that the pulsar is wobbling as it spins. In addition, it’s producing a comet-like tail behind it due to the effects of a pulsar wind nebula (PWN) — a sheath of high-energy particles that enshrouds the pulsar. And while a pulsar’s direction of motion is usually aligned with its jet and the pulsar wind nebula around it, IGR J11014-6103’s own PWN is almost perpendicular to the direction of the jet, possibly indicating high rotation speeds in the iron core of the supernova that produced the pulsar.
It’s fascinating to explore such extreme environments, and one of the things I love about science fiction is that a combination of scientific rigor and imagination can let us see things like this up close. Clement’s Mesklin was put together using a carefully wrought model that the author went on to describe in an article called ‘Whirligig World,’ which ran in Astounding Science Fiction in June of 1953. He based it on an object then believed to exist in the 61 Cygni system, and although the latter turned out to be a chimera, his concern for getting the science right built a memorable world we can still enjoy reading about today. Now who will take up Al Jackson’s challenge to describe a research station on a pulsar planet?
Image: The April, 1953 issue of Astounding contained the first installment of Hal Clement’s Mission of Gravity, published in book form the following year.
The paper on the light jet is Pavan et al. “The long helical jet of the Lighthouse nebula, IGR J11014-6103,” Astronomy & Astrophysics 562 (2014): A122 (preprint). Al Jackson’s paper on PSR 1257+12 is Malhotra et al. “Resonant orbital evolution in the putative planetary system of PSR1257 + 12,” Nature 356 (16 April 1992), pp. 583-585 (abstract).
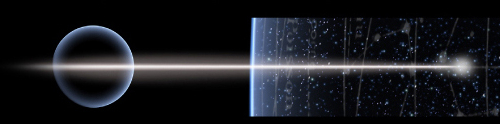
by Paul Gilster | Feb 25, 2014 | Exoplanetary Science |
Strong evidence for water in the atmosphere of the hot Jupiter Tau Boötis b has turned up, thanks to work by Geoffrey Blake (Caltech) and graduate student Alexandra Lockwood. But what’s intriguing about the find isn’t the water — we’ve found water vapor on other planets — but the method of detection. Lockwood and Blake used a modified radial velocity technique that has previously been deployed to detect low mass ratio binary stars. A top-flight instrument like the Near Infrared Echelle Spectrograph (NIRSPEC) at the W. M. Keck Observatory in Hawaii can separate the planetary and stellar components spectroscopically to produce this result.
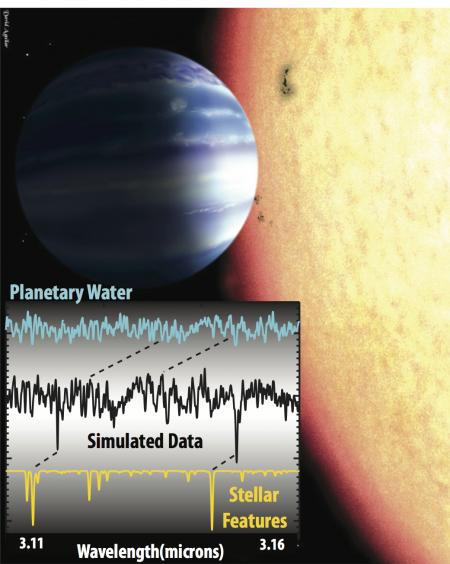
Image: Simulated data showing the method used for detecting water vapor features around the hot Jupiter tau Boötis b. In this example, the planetary signal has been increased in strength by several orders of magnitude relative to the actual signal. The dotted lines show the blue- and red-shifts of the planetary and stellar lines in the data, respectively. Credit: Alexandra Lockwood/Caltech.
Thus the toolkit of exoplanet science continues to grow. We already know how to study planetary atmospheres when a planet transits its primary as seen from Earth, allowing us to separate direct light from the star from light that has passed through the atmosphere. Another way of studying an atmosphere is through direct imaging, but here we rely on planets far enough from their host star that we can separate them from the star’s glare, the classic problem of planet hunters. But a non-transiting world like Tau Boötis b demands a different approach, and the spectroscopic separation of radial velocity signals appears to be one solution.
Lockwood is lead author of the paper on this work, which appears in The Astrophysical Journal Letters:
“The information we get from the spectrograph is like listening to an orchestra performance; you hear all of the music together, but if you listen carefully, you can pick out a trumpet or a violin or a cello, and you know that those instruments are present. With the telescope, you see all of the light together, but the spectrograph allows you to pick out different pieces; like this wavelength of light means that there is sodium, or this one means that there’s water.”
It was back in 2012 that two independent teams studying Tau Boötis b were able to separate the radial velocity of the planet from that of the star by studying shifts in the spectral lines of carbon dioxide. The technique as expanded into the infrared now gives us the molecular signature of water. I should mention here that a team in 2010 detected carbon monoxide in the atmosphere of another hot Jupiter, the much studied HD 209458 b, a detection that provided what researchers call the ‘spectroscopic orbit’ of the system and thus revealed the true mass of the planet.
So more is at play here than the detection of yet another component of a hot Jupiter’s atmosphere. Separating the two radial velocity components helps to determine a planet’s mass, which in earlier radial velocity studies could only be an estimate of the planet’s minimum mass. The actual mass could be much higher than what astronomers could measure, given their lack of knowledge about the inclination of the planet to the star. By detecting a signature from both planet and star, this method allows us to explore the system in greater detail. From the paper (with reference to the 2012 work on carbon dioxide mentioned above):
…this technique can be applied to non-transiting, RV-detected exoplanets to extract the unknown inclination and true mass. CRIRES [the High-Resolution IR Echelle Spectrometer installed at ESO’s Very Large Telescope] was also used to detect CO on τ Boötis b (Brogi et al. 2012), the first ground-based detection of a short-period non-transiting exoplanet atmosphere, a result confirmed shortly thereafter by Rodler et al. (2012). These studies provide the true mass of the planet and probe the chemical composition of its atmosphere. A combination of high signal-to-noise, high spectral resolution, and coverage of multiple CO overtone lines was required to achieve the sensitivity required for these detections.
So by finding the signature of water in the atmosphere of Tau Boötis b, the Caltech team is also extending work that creates what Lockwood calls the ‘3-D motion of the star and the planet in the system.’ It’s a method that deepens our understanding of the planetary system by giving us a true mass reading, and although at present its applications are limited to close-in gas giants like Tau Boötis b around nearby bright stars, the hope is that improvements in telescopes and infrared spectroscopy will extend the method to smaller planets around dimmer stars.
“While the current state of the technique cannot detect earthlike planets around stars like the Sun, with Keck it should soon be possible to study the atmospheres of the so-called ‘super-Earth’ planets being discovered around nearby low-mass stars, many of which do not transit,” adds Blake. “Future telescopes such as the James Webb Space Telescope and the Thirty Meter Telescope (TMT) will enable us to examine much cooler planets that are more distant from their host stars and where liquid water is more likely to exist.”
The paper is Lockwood et al. “”Near-IR Direct Detection of Water Vapor in tau Boötis b,” The Astrophysical Journal Letters, published online 24 February 2014 (preprint).
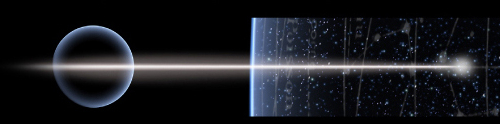
by Paul Gilster | Feb 24, 2014 | Exoplanetary Science |
CSIRO, the Commonwealth Scientific and Industrial Research Organisation in Australia, is announcing the detection of violent events around the pulsar PSR J0738-4042, some 37,000 light years from Earth in the constellation Puppis. This southern hemisphere constellation was originally part of a larger constellation called Argo Navis, depicting the ship made famous by the journey of Jason and the Argonauts. But Argo Navis was divided into three smaller constellations, leaving Puppis (The Stern) as something of a mythological fragment.
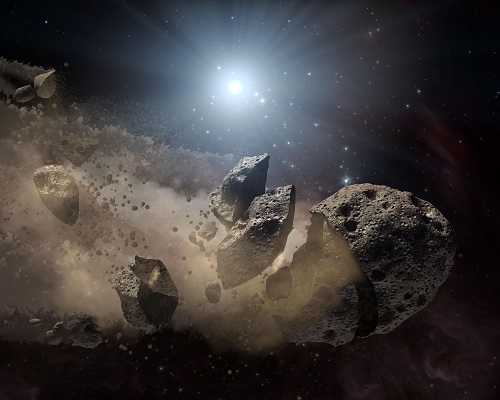
Image: An artist’s impression of an asteroid breaking up. Credit: NASA/JPL-Caltech.
Whatever its origins, Puppis is also home, from our Earthly perspective, to a pulsar around which radiation and sleeting high energy particles are common. Ryan Shannon, a member of the CSIRO research team, has previously examined how an infalling asteroid from a violent disk around a pulsar might affect it, slowing the pulsar’s spin rate and affecting the shape of the radio pulse that we see on Earth. That’s a useful signal, because pulsars flash a radio beam with great regularity, so that any disruptions call attention to themselves. Says Shannon:
“That is exactly what we see in this case. We think the pulsar’s radio beam zaps the asteroid, vaporising it. But the vaporised particles are electrically charged and they slightly alter the process that creates the pulsar’s beam.”
Long term monitoring has shown that the pulse shape of PSR J0738-4042 changes multiple times between 1988 and 2012, with one event that implies a rock of about a billion tonnes interacting with the pulsar. The abstract of the paper on this work summarizes the finding:
The torque, inferred via the derivative of the rotational period, changes abruptly from 2005 September. This change is accompanied by an emergent radio component that drifts with respect to the rest of the pulse. No known intrinsic pulsar processes can explain these timing and radio emission signatures.
And so we get an interesting mechanism for the formation of large objects around a pulsar. Dust and debris formed in the original stellar explosion that gave birth to the pulsar itself could fall back toward the spinning remnant to create a debris disk that, in turn, gives birth to larger objects. Recall that two planet-sized objects were detected in 1992 around the pulsar PSR 1257+12, though not necessarily formed by the same mechanisms (a third planet was detected five years later). We have much to learn about the various ways pulsar planets may form.
The environment that spawns these results has to give us pause. “If a large rocky object can form here,” Ryan Shannon adds, “planets could form around any star.” True enough, though we should add that pulsar planets still seem to be the rarest of the breed. In any case, this detection is remarkable. We’re reaching out to a distance far larger than that between the Earth and the galactic core to track conditions in this system. With so many ongoing projects, we can all too easily become blasé about just what is happening here, but let’s keep that sense of wonder at full throttle. Twenty-five years ago we had no confirmed exoplanets, and now we’re investigating asteroids in a system 37,000 light years from Earth? A golden era of discovery indeed…
The paper is Brook et al., “Evidence of an Asteroid Encountering a Pulsar,” Astrophysical Journal Letters Vol. 780, No. 2 (2014), L31 (abstract).
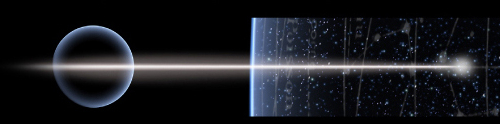