by Paul Gilster | Oct 31, 2018 | Exoplanetary Science |
The Kepler spacecraft has been with us long enough (it launched in 2009) and has revealed so much about the stars in our galaxy that its retirement — Kepler lacks the fuel for further science operations — is cause for reflection. The end of great missions always gives us pause as we consider their goals and their accomplishments, and offer up our gratitude to the many people who made the mission happen. Let’s try to back up and see things in their totality.
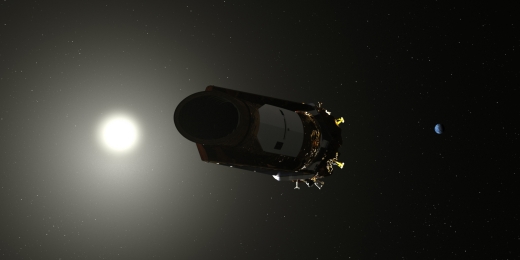
Image: An artist’s conception of Kepler at work. Credit: NASA/Ames/Dan Rutter.
Kepler’s job was essentially statistical, an attempt to look at as many stars as possible in a particular field of stars, so that we could gain insights into the distribution of planets there, and thus deduce something about likely conditions galaxy-wide. We didn’t know in 2009 that there was statistically at least one planet around every star, nor did we know just how diverse the worlds Kepler found, more than 2,600 of them, would be. Moreover, Kepler pushed the bounds of the possible with an ingenious extended mission.
After the mission was extended in 2012, engineers had to work around gyroscope failures to use solar photon pressure to keep the spacecraft pointed. The so-called ‘K2’ mission left the fixed view to move around the sky, studying 19 different patches of it and thus producing entirely different datasets. As with any mission, we’ve accumulated such extensive information that papers from Kepler data will be coming out for decades to come. Using the transit method to detect the passage of planets across the face of their star, Kepler’s planetary survey, originally studying over 156,000 stars, would move on through K2 to cover more than 500,000.
We have a great deal to learn as we carry Kepler discoveries forward, but it now seems likely that somewhere between 20 and 50 percent of all visible stars have rocky planets in the habitable zone of their stars. ‘Super Earths’ turned out to be the most common size of planet Kepler found, worlds between Earth and Neptune in size. Unless we find a hitherto undiscovered world deep in our outer system, we have no examples of such planets around Sol.
Interestingly, we’ve also learned how common compact planetary systems can be. Some of the Kepler systems bear more resemblance to Jupiter and its moons than planets and their host stars. KOI-961 is a case in point. About 130 light years away in Cygnus, the star is an M-dwarf about 70 percent larger than Jupiter, orbited by three small and likely rocky planets, all three of which orbit their host in less than two days.
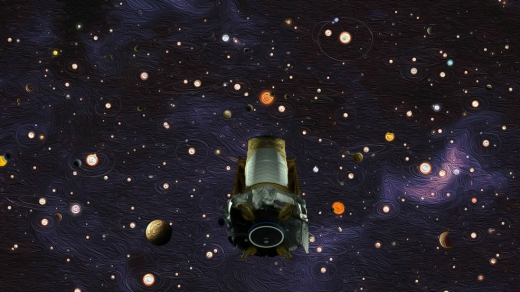
Image: This illustration depicts NASA’s exoplanet hunter, the Kepler space telescope. The agency announced on Oct. 30, 2018, that Kepler has run out of fuel and is being retired within its current and safe orbit, away from Earth. Kepler leaves a legacy of more than 2,600 exoplanet discoveries. Credit: NASA/Wendy Stenzel/Daniel Rutter.
Another Kepler find: Kepler-11, approximately 2000 light years away, with six planets, the outermost of which is twice as close to its star as the Earth is to the Sun. In fact, the five inner planets are closer to their star than Mercury is to the Sun. The list goes on: Kepler-32 is an M-dwarf with five transiting worlds orbiting within a distance that is just one-third the size of Mercury’s orbit. We now explore how such systems form and examine multi-planet systems like TRAPPIST-1 (not a Kepler discovery) that offer planets in the habitable zone.
Kepler also discovered its share of unusual exoplanets like Kepler-10b, a world with a year that lasts less than an Earth day and a density implying it is made of iron and rock, a ‘lava world.’ K2 found a planetary object around the white dwarf WD 1145+017. Kepler-16b is the first planet discovered around a double-star system, reminiscent of Tatooine in Star Wars. Kepler-444 has five planets that are 11 billion years old. And need I mention Boyajian’s Star, whose oddly fluctuating lightcurve is indicative most likely of dust clouds but was intensely studied in terms of possible alien engineering.
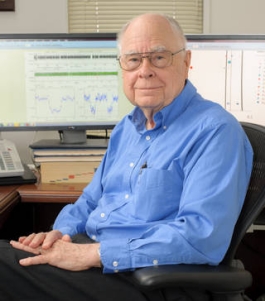
“When we started conceiving this mission 35 years ago we didn’t know of a single planet outside our solar system,” said the Kepler mission’s founding principal investigator, William Borucki, now retired from NASA’s Ames Research Center in California’s Silicon Valley. “Now that we know planets are everywhere, Kepler has set us on a new course that’s full of promise for future generations to explore our galaxy.”
Image: William Borucki, principal investigator for NASA’s Kepler mission, in his office at NASA’s Ames Research Center at Moffett Field, California. June 2015. Credit: NASA Ames.
Kepler kept sending us data even as it ran low on fuel, with the latest, from Campaign 19, marking a kind of handover to TESS, the Transiting Exoplanet Survey Satellite, which launched back in April. All told, we pulled in 678 GB of science data in Kepler’s 9.6 years in space, leading thus far to 2,946 published scientific papers, with many more to come. Last night at a favorite restaurant my wife and friends joined me in a toast to the Kepler team, and the mission’s indefatigable PI William Borucki. An outstanding accomplishment all around.
To wrap this up, here’s Kepler’s ‘first light’ image from 2009.
Image: This image from NASA’s Kepler mission shows the telescope’s “first light” — a full field of view of an expansive star-rich patch of sky in the constellations Cygnus and Lyra stretching across 100 square degrees, or the equivalent of two side-by-side dips of the Big Dipper. Credit: NASA.
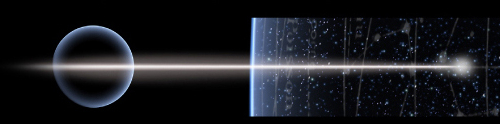
by Paul Gilster | Oct 29, 2018 | Outer Solar System |
The interstellar object called ‘Oumuamua continues to inspire analysis and speculation. And no wonder. We had limited time to observe it and were unable to obtain a resolved image to find out exactly what it looks like. This morning I want to go through a new paper from Shmuel Bialy and Abraham Loeb (Harvard University) considering the role radiation pressure from the Sun could play on this deep sky wanderer. Let’s also review what we do know about it, which I’ll do with reference to this paper’s introduction, where recent work is discussed. For it seems that each time we look at ‘Oumuamua anew, we find something else to talk about.
Discovered in October of 2017 by the Pan-STARRS survey (Panoramic Survey Telescope and Rapid Response System) in Hawaii, ‘Oumuamua stood out because of its hyperbolic trajectory, flagging it as an interstellar object, the first ever discovered passing through the Solar System. The object’s lightcurve indicated both that it was tumbling and had an aspect ratio of at least 5:1 and perhaps higher, an unusual shape for known asteroids and comets. I won’t provide all the references here, as they’re easily found both in the paper cited below and in other related work.
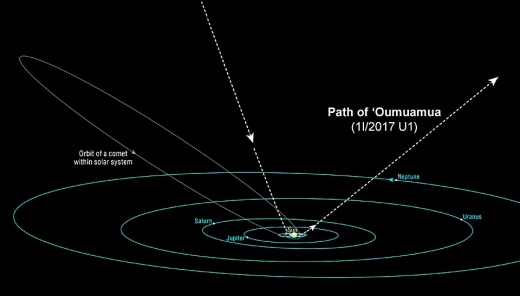
Image: The track of `Oumuamua as it passed through the inner solar system in late 2017. Credit: Brooks Bays / SOEST Publication Services / Univ. of Hawaii.
What stood out in 2018 was detection of non-gravitational acceleration in ‘Oumuamua’s motion, which could be consistent with cometary activity, although as we’ve seen in other posts on this site, no such activity has been noted by way of a cometary tail or gas emission and absorption lines. This despite a relatively close approach to the Sun of 0.25 AU. The Micheli et al. paper noting the acceleration was addressed in another 2018 paper from Rafikov et al., which pointed out that torque from any cometary outgassing should have had effects on the object’s spin, but this does not appear in our admittedly limited observations.
What Bialy and Loeb consider in today’s paper is the possibility that solar radiation pressure — imparted by the momentum of photons from the Sun — is responsible for the acceleration, expressed as an excess radial acceleration ?a ? r?2, where r is the distance of ’Oumuamua from the Sun. If so, ‘Oumuamua would of necessity be a thin object with a small mass-to-area ratio — this is required in order to make the radiation pressure effective.
We can work out constraints on the object’s area through its observed magnitude. The paper proceeds to show that a thin sheet roughly 0.3 mm thick and some 20 meters in radius will allow the non-gravitational acceleration computed in the Micheli paper.
I was intrigued enough at this point to ask Dr. Loeb about those dimensions, which vary with albedo (the incident light reflected by a surface). He told me that the 20-meter figure would be the radius if the object is a perfect reflector, though the size would be larger if the value for the albedo is smaller. We do see variations in reflected light as ‘Oumuamua rotates over an eight-hour spin period. Thus, considering the object as a thin surface, we could imagine a conical or hollow cylindrical shape. “You can easily envision that by rotating a curved piece of paper and looking at its net surface area from different viewing angles,” Loeb told me.
So let’s back up a moment. We are asking what properties ‘Oumuamua would have to have if its non-gravitational acceleration is the result of solar radiation pressure. We do not know that solar radiation is the culprit, but if it is, the object would need to be a thin sheet with a width in the range of 0.3 mm. This scenario explains the acceleration but forces the question of what kind of object could have these characteristics. A major problem is that, as mentioned above, there are too many degrees of freedom in our observations to nail down what ‘Oumuamua looks like. We did not have observations sensitive enough to produce a resolved image.
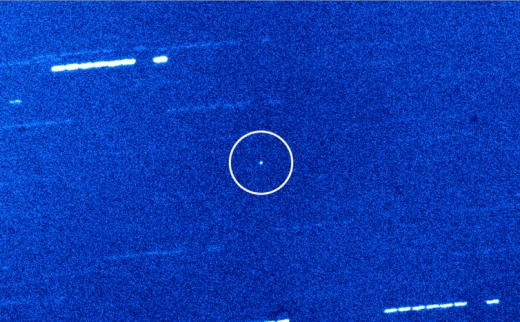
Image: Oumuamua as it appeared using the William Herschel Telescope on the night of October 29. Credit: Queen’s University Belfast/William Herschel Telescope.
We do know that if ‘Oumuamua is accelerating because of solar photons, it must represent what the paper calls ‘a new class of thin interstellar material.’ The researchers note the possibility that such material is naturally produced in the interstellar medium, but go on to consider an artificial origin. Could ‘Oumuamua be debris from a technological civilization, a discarded lightsail?
A fascinating speculation indeed. From the paper:
Considering an artificial origin, one possibility is a lightsail floating in interstellar space as debris from an advanced technological equipment (Loeb 2018). Lightsails with similar dimensions have been designed and constructed by our own civilization, including the IKAROS project and the Starshot Initiative. The lightsail technology might be abundantly used for transportation of cargos between planets (Guillochon & Loeb 2015) or between stars (Lingam & Loeb 2017). In the former case, dynamical ejection from a planetary system could result in space debris of equipment that is not operational any more (Loeb 2018) and is floating at the characteristic speed of stars relative to each other in the Solar neighborhood.
We’re past the stage where we can image ‘Oumuamua with our telescopes and it’s too late to get a mission off to chase it with chemical rockets, which means that the only way we have of pressing the investigation forward is to look for other such objects in the future. But we can look at the properties of thin films to determine whether an object like a lightsail could survive interstellar travel, given encounters with dust and gas between the stars. Returning to the paper:
Collisions with dust grains at high velocities will induce crater formation by melting and evaporation of the target material. Since the typical time between dust collisions is long compared to the solidification time, any molten material will solidify before the next collision occurs, and thus will only cause a deformation of the object’s surface material, not reduction in mass. On the other hand, atoms vaporized through collisions can escape and thus cause a mass ablation.
Bialy and Loeb find that for the mass-to-area ratio they have calculated for a thin-film ‘Oumuamua, the object could travel through much of the galaxy before losing a significant fraction of its mass. Collisions with gas particles in the interstellar medium as well as the stresses of centrifugal and tidal forces are also considered. None of these present problems for the object’s survival until we reach a maximal travel distance in the range of 10 kpc (well over 32,000 light years). Earth is approximately 25,000 light years from the center of the galaxy.
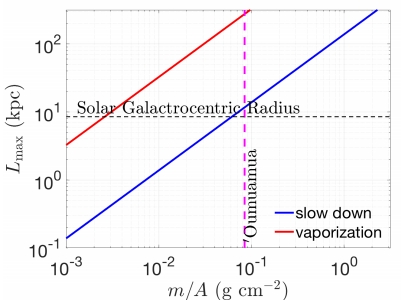
Image: This is Fig. 1 from the paper. Caption: The maximum allowed travel distance through the interstellar medium (ISM), as a function of (m/A). The blue and red lines are limitations obtained by slow-down due to gas accumulation, and vaporization by dust-collisions, respectively. The plotted results are for a mean ISM proton density of (n) ? 1 cm?3. All lines scale as 1/(n). The dashed magenta line is our constraint on ’Oumuamua based on its excess acceleration. The Solar Galactrocentric distance is also indicated. Credit: Bialy & Loeb.
Avi Loeb has recently written about how we might find evidence for extraterrestrial civilizations long gone. From that perspective, he considered the possibility that ‘Oumuamua is just such an artifact, and told me this in an email:
With the perspective of my recent essay in Scientific American, `Oumuamua could be defunct sails floating under the influence of gravity and stellar radiation. Similar to debris from ship wrecks floating in the ocean. The alternative is to imagine that `Oumuamua was on a reconnaissance mission. The reason I contemplate the reconnaissance possibility is that the assumption that `Oumumua followed a random orbit requires the production of ~1015 such objects per star in our galaxy. This abundance is up to a hundred million times more than expected from the Solar System, based on a calculation that we did back in 2009. A surprisingly high overabundance, unless `Oumuamua is a targeted probe on a reconnaissance mission and not a member of a random population of objects.
Loeb also made a comment in his email that ties in to what Breakthrough Starshot is attempting to quantify, a series of missions to the same target — hundreds if not thousands of probes — sent swarm-like to ensure that at least one or a few come close to the world under observation. If something like this were happening with ‘Oumuamua, and given that PAN-STARRS barely detected the object at closest approach, we would not know about any of its fellow probes.
Addendum: Just as I was publishing this I learned of a paper by Eric Mamajek (University of Rochester), who notes that ‘Oumuamua appears to have originated at the Local Standard of Rest (LSR), which is the galactic frame of reference. Quoting Mamajek: “Compared to the LSR, ‘Oumuamua has negligible radial and vertical Galactic motion…” According to Dr. Loeb in a subsequent email, less than one star in 500 is at that frame of reference to the same precision. (The Mamajek paper, “Kinematics of the Interstellar Vagabond 1I/’Oumuamua (A/2017 U1),” can be found here in preprint form).
It’s an interesting point. If ‘Oumuamua turned out to be artificial, would there be an advantage in such a position? Perhaps so, for as Loeb goes on to say in his email:
I view a sail (like `Oumuamua) floating in interstellar space with stars (like the Sun) running into it as if it were a buoy floating on the ocean surface with boats colliding with it. An artificial origin would naturally place floating sails at the LSR, perhaps as relay stations.
Another item of interest:
Trilling et al., “Spitzer observations of `Oumuamua and `Oumuamua’s density and shape,” as presented at the American Astronomical Society DPS meeting #50, finds no detection of thermal emission from `Oumumua, implying that it must be very reflective or small, which is consistent with the Bialy and Loeb paper. The abstract of the Trilling paper is here.
And a final point: While catching up with ‘Oumuamua would be immensely challenging, Andreas Hein and colleagues have suggested how it might be done. For more, see Project Lyra: Sending a Spacecraft to 1I/’Oumuamua (formerly A/2017 U1), the Interstellar Asteroid.
The Bialy & Loeb paper is “Could Solar Radiation Pressure Explain ‘Oumuamua’s Peculiar Acceleration?” (preprint). The Micheli et al. paper on ‘Oumuamua’s acceleration is “Non-gravitational acceleration in the trajectory of 1I/2017 U1 (‘Oumuamua),” Nature 559 (27 June 2018), 223-226 (abstract).
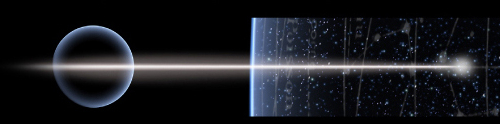
by Paul Gilster | Oct 26, 2018 | Asteroid and Comet Deflection |
With two rovers and a lander already deployed on the asteroid 162173 Ryugu, the Japan Aerospace Exploration Agency (JAXA) must be basking in the glow of an unusually successful venture. Now we turn to a key part of the Hayabusa2 mission, the retrieval of a surface sample. Two touchdown rehearsals have gone well, providing detailed views of the asteroid’s surface. The plan is to return samples to Earth in December of 2020, but let’s continue to take one thing at a time. Sample retrieval can be dicey, as we saw with the first Hayabusa.
Once known as MUSES-C, the original Hayabusa reached asteroid Itokawa in September of 2005 (I can’t believe it was that long ago — as the cliché would have it, it seems like yesterday). A series of enroute problems included a solar flare that damaged the craft’s solar cells and the failure of attitude-adjusting reaction wheels, while the launch of a probe called MINERVA also failed. Nonetheless, surface particles from Itokawa were successfully returned to Earth.
Now the Hayabusa2 team works on landing site selection, no easy task. Keeping the spacecraft safe means looking for regions ideally 100 meters in diameter in which there is an average slope of less than 30 degrees, boulder heights less than 50 centimeters, and an absolute temperature less than 370 Kelvin (97 degrees Celsius). These constraints limit the site to 30 degrees either side of the asteroid’s equator, all this on a diminutive object that presents its own problems.
“Unlike other asteroids we have visited, Ryugu has no powder, no fine-grain regolith. That makes selecting a place to sample more challenging,” said the Planetary Science Institute’s Deborah Domingue. “We are helping characterize the surface to optimize landing site selection.”
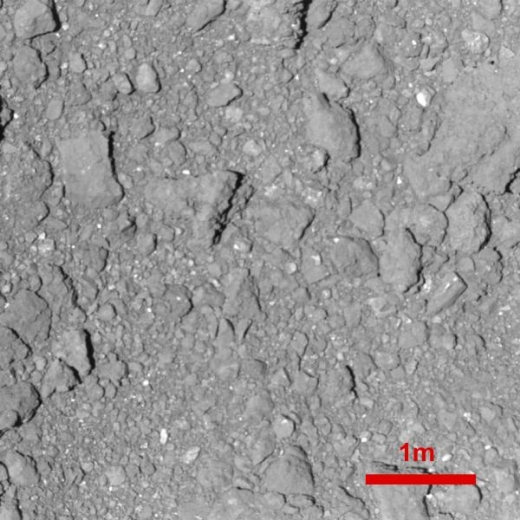
Image: This image of asteroid Ryugu was taken with Hayabusa2’s ONC-T (Optical Navigation Camera-Telescopic) on October 15, 2018 from an altitude of 42 meters. The resolution is about 4.6 millimeters per pixel, and this is the highest resolution that Hayabusa2 spacecraft has taken. In fact, this is the highest resolution image that a spacecraft has taken of an asteroid. Credit: JAXA, Tokyo University, Kochi University, Rikkyo University, Nagoya University, Chiba Institute of Technology, Meiji Univeristy, Aizu University, and AIST.
Along with PSI’s Lucille Le Corre, a co-investigator on the mission’s Optical Navigation Camera (ONC) team, Domingue discussed these and other Hayabusa2 operations at a press conference at the 50th annual meeting of the American Astronomical Society’s Division for Planetary Sciences (DPS) in Knoxville. Hayabusa2 team members present included Masaki Fujimoto, the head of ISAS/JAXA, Hikaru Yabuta, lead of the landing site selection committee and the multi-scale regolith characterization team, Ralf Jaumann, lead for the European Space Agency’s (ESA’s) MASCOT lander, and Eri Tatsumi, the ONC team instrument scientist.
“Since the approach phase began last June, my main goal was to support the Hayabusa2 team in the preparation of touch down operations,” said Le Corre. “In order to assess the sampleability of Ryugu’s terrains I have worked on generating products such as ONC image mosaics and local topographic models. Our data show that the Hayabusa2 team has to carefully select a sampling site to avoid the numerous boulders present on the surface.”
Lacking fine-grained regolith, Ryugu is challenging, and the mission demands a thorough analysis of the surface to ensure a successful sample collection. Three sample collections are planned, the first now likely no sooner than January of 2019. The first two sample collections will use a sampler horn that will catch particles of ejecta raised by the firing of a projectile into the surface. The third sample collection, in the late spring, will collect material dislodged by a kinetic impactor that will produce a crater on the surface of Ryugu.
The image below (taken by a camera that, incidentally, was funded by public contributions) comes from the Hayabusa2 website and shows the sampler horn.
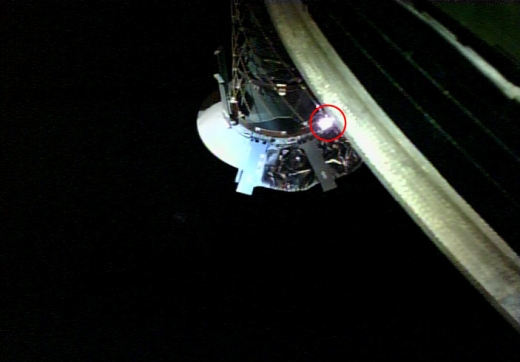
Image: Test photograph of the sampler horn taken by the Small Monitor Camera (CAM-H) on April 16, 2018. The shiny part in the red circle is the target plate of the sampler horn which is illuminated by the LRF-S2 laser. If the laser misses this plate, touchdown is in process and bullets will fire to stir up surface material. Credit: JAXA.
The LRF-S2 laser referred to in the caption is a part of planned sample collection activities. In the image, the LRF-S2 laser beam is hitting the tip of the sampler horn, indicating the craft is ready for touchdown operations. During touchdown, the sampler horn will compress as it touches the surface. JAXA describes the operation this way:
When the distance measurement from LRF-S2 changes or the laser from LRF-S2 deviates from its target at the base of the sampler horn, bullets will be fired to stir up the surface material for collection. This makes the LRF-S2 an important device for successful sample collection.
To keep up with Hayabusa2 operations, keep an eye on Twitter @haya2e_jaxa, from which I drew the tweet below.
And here is the image with possible touchdown area LO8-B, considered the most promising candidate.
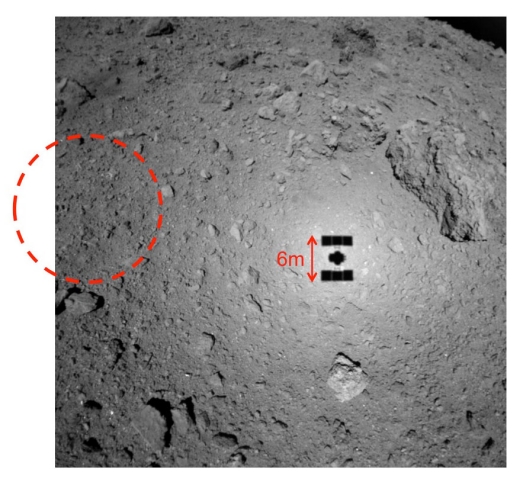
Image: The surface of Ryugu captured with the ONC-W1 at an altitude of about 47m. The image was taken on October 15, 2018 at 22:45 JST. The red circle indicates the candidate point for touchdown, L08-B. Credit: JAXA, University of Tokyo, Kochi University, Rikkyo University, Nagoya University, Chiba Institute of Technology, Meiji University, University of Aizu, AIST).
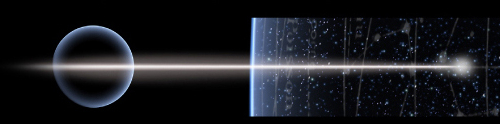
by Paul Gilster | Oct 25, 2018 | Outer Solar System |
To say that the Space Science and Engineering Division at Southwest Research Institute has been busy of late is quite an understatement. Alan Stern, principal investigator for New Horizons, has been leading an SwRI study examining just how we might operate an orbiter at Pluto/Charon, with results that are surprising and encouraging for the future of such a project.
Working with spaceflight engineer and mission designer Mark Tapley and planetary scientist Amanda Zangari, as well as project manager John Scherrer and software lead Tiffany Finley, Stern has been looking at an orbital tour of Pluto built around a series of gravity assist maneuvers involving Charon, its large moon. The mission would use the kind of electric propulsion system we saw in the Dawn mission to Vesta and Ceres, and by clever use of gravity assists, would pull off another Dawn feat by leaving Pluto once its orbital operations were concluded and moving into the Kuiper Belt for encounters with further objects.
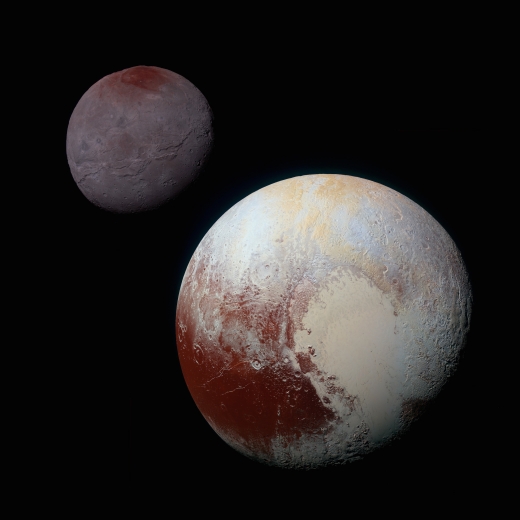
Image: NASA’s New Horizons mission made the first exploration of Pluto-Charon and its system of small moons in 2015. The scientific discoveries led the science community to push for a return to Pluto with an orbiter or to explore other dwarf planets in the Kuiper Belt. Credit: NASA/JHUAPL/SwRI.
The news comes out of a workshop on Pluto and Kuiper Belt exploration held at the American Astronomical Society’s Division for Planetary Sciences meeting in Knoxville, Tennessee. Gravity assists from Charon save propellant, making it possible to continually change the orbiter’s track over Pluto while studying its surface and atmosphere, as well as its system of moons. Several years in such an adjustable orbit would allow us to home in on major discoveries from New Horizons while also uncovering numerous other features that spacecraft did not see.
But it’s also the move into the Kuiper Belt that has Stern’s attention. It makes a Pluto orbiter of this design a multi-purpose spacecraft and leverages our growing experience with ion propulsion. Says Stern:
“This is groundbreaking. Previously, NASA and the planetary science community thought the next step in Kuiper Belt exploration would be to choose between ‘going deep’ in the study of Pluto and its moons or ‘going broad’ by examining smaller Kuiper Belt objects and another dwarf planet for comparison to Pluto. The planetary science community debated which was the right next step. Our studies show you can do both in a single mission: it’s a game changer.”
As analyzed by Tiffany Finley, the orbital tour, still being optimized, would allow dozens of gravity assists at Charon, offering a series of flybys of Pluto’s smaller moons as well as close encounters with Charon itself. Finley talks about studying Pluto’s polar and equatorial regions using plane changes enabled by these maneuvers, including sampling the dwarf planet’s atmosphere. A final Charon gravity assist would take the orbiter into the Kuiper Belt.
And Mark Tapley’s work on electric propulsion ups the ante. He’s arguing that not only would this craft be able to perform flybys of Kuiper Belt objects, but that it could even enter orbit around a second dwarf planet in the Kuiper Belt, just as Ceres followed Vesta for Dawn.
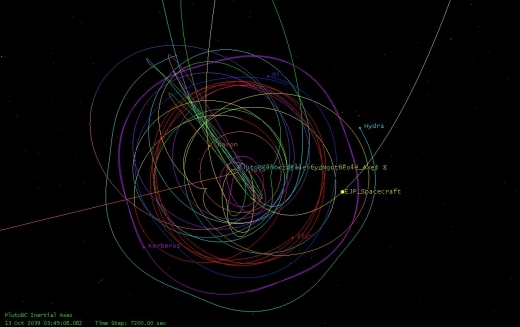
Image: The SwRI-developed Pluto orbital tour design shows scientific objectives can be met with only tiny fuel resources and numerous gravity assists using Pluto’s giant moon Charon. Credit: Southwest Research Institute.
What sort of missions are we talking about? Amanda Zangari’s separate study for this group has looked at the 45 largest Kuiper Belt objects and dwarf planets (covering “the most desirable and scientifically interesting targets”) with launches possible between 2025 and 2040. How these trajectories might be accomplished is presented in a paper that has been accepted by the Journal of Spacecraft and Rockets. From the paper, this is a bit of an eye-opener:
There is a KBO mission possible for every Earth-Jupiter launch window throughout a Jupiter revolution, thus Pluto and every one of the selected 45 KBOs are accessible via Jupiter gravity assist with a flight time of under 25 years and a C3 [excess launch energy] less than 140 km2/s2. Many, but not all objects can be reached via Saturn flyby, and a smaller list still can be compatible with a visit to an ice giant, though it does not necessarily provide a TOF [time of flight] advantage.
Which leads to this:
We found that all five of the non-Pluto KBOs studied by McGranaghan et al [23] can be reached by giant planet swingby— (136199) Eris and (90377) Sedna with Neptune, and (50000) Quaoar, (136472) Makemake and (136108) Haumea via Jupiter-Saturn. Fast-rotator (20000) Varuna is reachable after a Uranus encounter.
Working out the best targets will be critical, for as the paper goes on to note:
We have not considered multiple KBO flybys. It is worth noting that the prospect of an additional flyby of a KBO after Pluto played a large role in New Horizons’ selection, and that it was possible because of Pluto’s proximity at the time to the plane of the Solar System. Many of our KBOs have highly inclined orbits and are often far outside the plane of the Solar System at the time of flyby, thus no additional flybys will be possible en route owing to the vast separations between objects. To probe a second KBO typically requires one to fly through the classical belt.
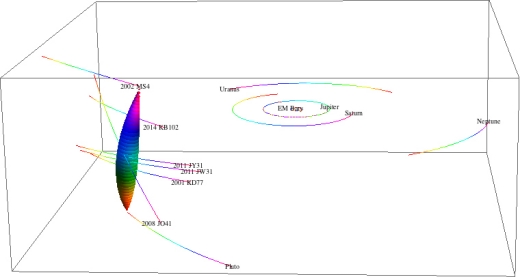
Image: This figure depicts an electric propulsion spacecraft leaving Pluto orbit, flying by other small Kuiper Belt objects and then reaching the dwarf planet 2002 MS4 as just one example of the “gold standard” Pluto orbiter-Kuiper Belt explorer mission discovered by the SwRI study team. Credit: Southwest Research Institute.
We’ll need a good name for the Pluto orbiter/Kuiper Belt explorer mission, so start thinking about it now as we begin hoping that such a mission will be funded. Back to Stern for the conclusion:
“Who would have thought that a single mission using already available electric propulsion engines could do all this? Now that our team has shown that the planetary science community doesn’t have to choose between a Pluto orbiter or flybys of other bodies in the Kuiper Belt, but can have both, I call this combined mission the ‘gold standard’ for future Pluto and Kuiper Belt exploration.”
The team’s paper on spacecraft trajectories at Pluto/Charon and beyond is Zangari et al., “Return to the Kuiper Belt: launch opportunities from 2025 to 2040,” accepted at the Journal of Spacecraft & Rockets (preprint).
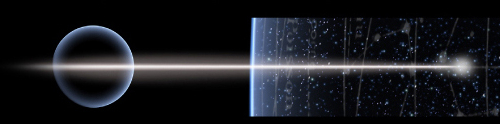
by Paul Gilster | Oct 24, 2018 | Outer Solar System |
Europa stays in this news this morning as we continue to correlate recent observations with the invaluable results of the Galileo mission. Hubble data have played a role in this, with researchers identifying plume activity in 2013 that recalled the geysers of Enceladus, a possible indication of venting from the subsurface ocean. But analysis of Cassini data from its 2001 Jupiter flyby enroute to Saturn showed no evidence of plume activity through its ultraviolet imaging spectrograph (UVIS).
So what exactly did Hubble see? Yesterday’s post highlighted Julie Rathbun’s contention that if they are there, Europan plumes show no thermal signature in Galileo data, while Xianzhe Jia (University of Michigan) and the SETI Institute’s Melissa McGrath have used Galileo magnetometer data to support possible plume activity. We may need Europa Clipper to resolve the matter.
Now the 66 dish antennas of the Atacama Large Millimeter/submillimeter Array (ALMA) have been turned on Europa in an attempt to study the entire moon — not just the areas of possible plume activity — to develop a thermal model. The data allow us to find what the authors of the paper on this work describe as “significant thermal structure.” What we get out of this is the ability to construct a global map showing the varying heat signatures across this remote world, in the process revealing an unusual cold spot on Europa’s northern hemisphere.
“These ALMA images are really interesting because they provide the first global map of Europa’s thermal emission,” said Samantha Trumbo, a planetary scientist at the California Institute of Technology and lead author on a paper published in the Astronomical Journal. “Since Europa is an ocean world with potential geologic activity, its surface temperatures are of great interest because they may constrain the locations and extents of any such activity.”
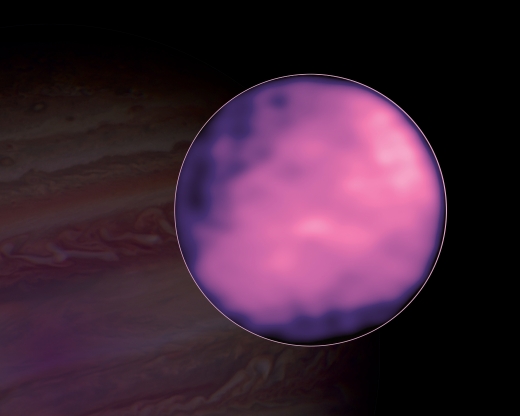
Image: ALMA image of Jupiter’s moon Europa. ALMA was able to map out thermal variations on its surface. Hubble image of Jupiter in the background. Credit: ALMA (ESO/NAOJ/NRAO), S. Trumbo et al.; NRAO/AUI NSF, S. Dagnello; NASA/Hubble.
ALMA’s ability to detect Europa’s thermal ‘glow’ is remarkable given that its surface temperature is never warmer than about minus 160 degrees Celsius, but this is an instrument that can provide insights into not just Jovian moons but asteroids and comets as well. On Europa, ALMA’s images have a resolution of 200 kilometers. Learning more about Europa’s surface can give us insights into its geological processes, especially interesting given that this surface is comparatively young, an indication of thermal or geological activity that has yet to be identified.
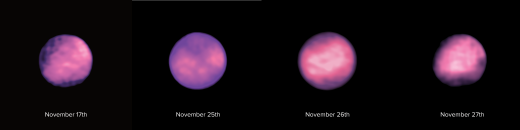
Image: Series of 4 images of the surface of Europa taken with ALMA, enabling astronomers to create the first global thermal map of Jupiter’s icy moon. Credit: ALMA (ESO/NAOJ/NRAO), S. Trumbo et al.
Trumbo and colleagues developed their global thermal model of Europa based on the ALMA observations, folding in an albedo map constructed from Voyager measurements and factoring in Galileo data. As for that odd cold spot on Europa’s northern hemisphere, it stands out on the ALMA maps near 90 degrees West and 23 degrees North. It also appears in two different images at two different times of day, an indication that it is genuinely distinct in its thermal properties. Moreover, Galileo may not be able to help us here, as the paper explains:
This region coincides with the location of highest water ice abundance mapped by Brown & Hand (2013) and the location suggested to have the most crystalline water ice by Ligier et al. (2016). However, it does not correspond to any particular geologic unit (Leonard et al. 2017) or any unusual visible morphological or albedo features (USGS 2002). The anomaly is located within a region of relatively low-resolution imaging and was not covered by the published Galileo PPR [Photopolarimeter-Radiometer] data, so it is possible that a corresponding morphological, geologic, or thermal feature was simply not seen by Galileo.
What to make of it? Except for discrepancies like this one, the model the authors work with reproduces the thermal structure seen by ALMA, an indication that we are seeing the results of albedo variation across the surface and the absorption and re-emission of sunlight. However, they find little correlation between the heat signatures and surface geology except for the area around Pwyll crater (this is similar to crater ejecta data elsewhere in the Solar System, which also show a distinctive signature). The cold spot referenced above remains enigmatic and will await future ALMA observations.
An afterword on Pwyll crater, which has been associated with possible plume activity: The ALMA map shows that Pwyll has a “locally elevated thermal inertia.” What this means is that this area takes longer to reach equilibrium with local temperature change than areas around it. Various factors are at play here, including density and thermal conductivity. Of particular interest is that the ‘cold spot’ anomaly is almost directly on the other side of Europa from Pwyll.
There is an analogy here with our own Moon, and the association with geological features on it that are directly opposite, or antipodal, to Tycho crater. But that is about as far as we can go with existing data. “Unfortunately,” the authors write, “without new, high-resolution imaging, the potential association of this feature with unique geology will likely remain an open question.”
The paper is Trumbo et al., “ALMA Thermal Observations of Europa,” Astronomical Journal Vol. 156, No. 4 (18 September 2018). Abstract / Preprint.
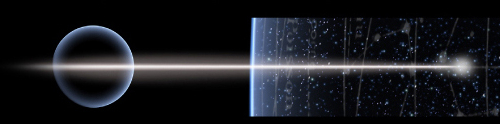