by Paul Gilster | Jun 30, 2017 | Astrobiology and SETI |
I came across the work of Chin-Fei Lee (Academia Sinica Institute of Astronomy and Astrophysics, Taiwan) when I had just read Avi Loeb’s essay Cosmic Modesty. Loeb (Harvard University) is a well known astronomer, director of the Institute for Theory and Computation at the Harvard-Smithsonian Center for Astrophysics and a key player in Breakthrough Starshot. His ‘cosmic modesty’ implies we should accept the idea that humans are not intrinsically special. Indeed, given that the only planet we know that hosts life has both intelligent and primitive lifeforms on it, we should search widely, and not just around stars like our Sun.
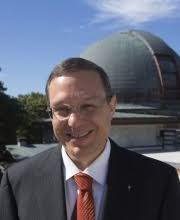
More on that in a moment, because I want to intertwine Loeb’s thoughts with recent work by Chin-Fei Lee, whose team has used the Atacama Large Millimeter/submillimeter Array (ALMA) to detect organic molecules in an accretion disk around a young protostar. The star in question is Herbig-Haro (HH) 212, an infant system (about 40,000 years old) in Orion about 1300 light years away. Seen nearly edge-on from our perspective on Earth, the star’s accretion disk is feeding a bipolar jet. This team’s results, to my mind, remind us why cosmic modesty seems like a viable course, while highlighting the magnitude of the question.
Image: Harvard’s Avi Loeb. Credit: Harvard University.
What Lee’s team has found at HH 212 is an atmosphere of complex organic molecules associated with the disk. Methanol (CH3OH) is involved, as is deuterated methanol (CH2DOH), methanethiol (CH3SH), and formamide (NH2CHO), which the researchers see as precursors for producing biomolecules like amino acids and sugars. “They are likely formed on icy grains in the disk and then released into the gas phase because of heating from stellar radiation or some other means, such as shocks,” says co-author Zhi-Yun Li of the University of Virginia.
Every time I read about finds like this, I think about the apparent ubiquity of life’s materials — here we’re seeing organics at the earliest phases of a stellar system’s evolution. The inescapable conclusion is that the building blocks of living things are available from the outset to be incorporated in the planets that emerge from the disk. That certainly doesn’t count as a detection of life, but it does remind us of how frequently the ingredients of life manage to appear.
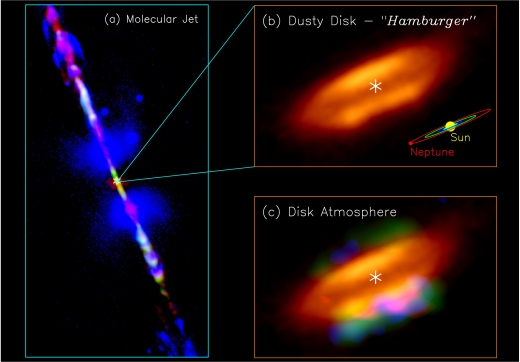
Image: Jet, disk, and disk atmosphere in the HH 212 protostellar system. (a) A composite image for the HH 212 jet in different molecules, combining the images from the Very Large Telescope (McCaughrean et al. 2002) and ALMA (Lee et al. 2015). Orange image shows the dusty envelope+disk mapped with ALMA. (b) A zoom-in to the central dusty disk. The asterisk marks the position of the protostar. A size scale of our solar system is shown in the lower right corner for comparison. (c) Atmosphere of the accretion disk detected with ALMA. In the disk atmosphere, green is for deuterated methanol, blue for methanethiol, and red for formamide. Credit: ALMA (ESO/NAOJ/NRAO)/Lee et al.
In that context, Avi Loeb’s thoughts on cosmic modesty ring true. We’ve been able to extract some statistical conclusions from the Kepler instrument’s deep stare that let us infer there are more Earth-mass planets in the habitable zones of their stars in the observable universe than there are grains of sand on all the Earth’s beaches. Something to think about as you read this on your beach vacation and gaze from the sand beneath your feet to the ocean beyond.
But are most living planets likely to occur around G-class stars like our Sun? Loeb reminds us that red dwarf stars like Proxima Centauri b and TRAPPIST-1, both of which made headlines in the past year because of their conceivably habitable planets, are long-lived, with lifetimes as long as 10 trillion years. Our Sun’s life, by comparison, is a paltry 10 billion years. Long after the Sun has turned into a white dwarf after its red giant phase, living things could still have a habitat around Proxima Centauri and TRAPPIST-1. Says Loeb:
I therefore advise my wealthy friends to buy real estate on Proxima b, because its value will likely go up dramatically in the future. But this also raises an important scientific question: “Is life most likely to emerge at the present cosmic time near a star like the sun?” By surveying the habitability of the universe throughout cosmic history from the birth of the first stars 30 million years after the big bang to the death of the last stars in 10 trillion years, one reaches the conclusion that unless habitability around low-mass stars is suppressed, life is most likely to exist near red dwarf stars like Proxima Centauri or TRAPPIST-1 trillions of years from now.
But of course, one of the reasons for missions like TESS (Transiting Exoplanet Survey Satellite) is to begin to understand the small rocky worlds around nearby red dwarfs, and to determine whether there are factors like tidal lock or stellar flaring that preclude life there. For that matter, do the planets around Proxima and TRAPPIST-1 have atmospheres? There too the answer will be forthcoming, assuming the James Webb Space Telescope is deployed successfully and can make the needed assessment of these worlds.
…very advanced civilizations [Loeb continues] could potentially be detectable out to the edge of the observable universe through their most powerful beacons. The evidence for an alien civilization might not be in the traditional form of radio communication signals. Rather, it could involve detecting artifacts on planets via the spectral edge from solar cells, industrial pollution of atmospheres, artificial lights or bursts of radiation from artificial beams sweeping across the sky.
Changes to the traditional view of SETI abound as we explore these new pathways. In any case, our technologies for making such detections have never been as advanced, and work across the exoplanetary spectrum, such as the findings of Chin-Fei Lee and colleagues, urges us on as we try to relate our own civilization to a universe in which it is hardly the center. As Loeb reminds us, we are orbiting a galaxy that itself moves at ~0.001c relative to the cosmic rest frame, one of perhaps 100 billion galaxies in the observable universe.
Either alternative — we are alone, or we are not — changes everything about our perspective, and encourages us to deepen the search for simple life (perhaps detected in exoplanetary atmospheres through its biosignatures) as well as conceivable alien civilizations. Embracing Loeb’s cosmic modesty, we press on under the assumption that life’s emergence is not uncommon, and that refining the search to learn the answer is a civilizational imperative.
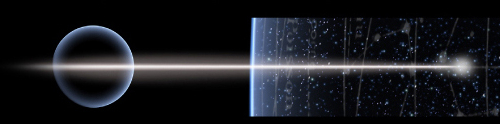
by Paul Gilster | Jun 29, 2017 | Outer Solar System |
Perhaps the image of Uranus just below helps explain why the planet has been treated so sparsely in science fiction. Even this Voyager view shows us a featureless orb, and certainly in visible light the world has little to make it stand out other than its unusual axis of rotation, which is tilted so that its polar regions are where you would expect its equator to be. Geoff Landis’ “Into the Blue Abyss” (2001) is the best fictional treatment I know, but the fog-shrouded Uranus of Stanley G. Weinbaum’s “The Planet of Doubt” (1935) has its own charms, though obviously lacking the scientific verisimilitude of the Landis tale.
My admiration for Gerald Nordley’s “Into the Miranda Rift” (1993) is unabated, taking us into this strange world’s most dramatic moon, while I should also mention Kim Stanley Robinson’s visit to Uranus in Blue Mars (1997), where the moon is established as a protected wilderness site while the rest of the Uranian satellite system is under colonization. Fritz Leiber’s “Snowbank Orbit” (1962) explores aero-braking at Uranus, while Larry Niven’s A World Out of Time (1976) maneuvers the planet to adjust the Earth’s own orbit.
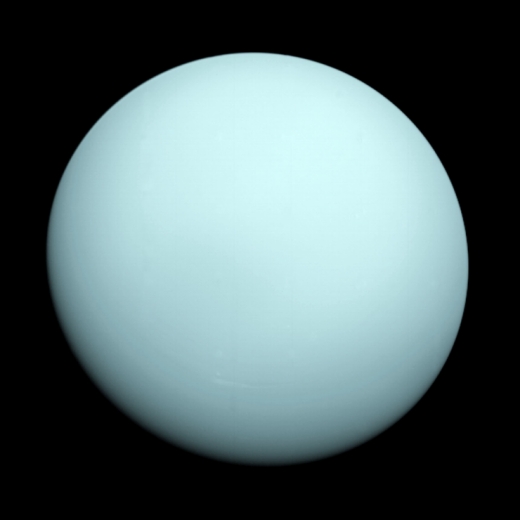
Image: The planet Uranus as seen by Voyager 2, which flew closely past the seventh planet in January of 1986. Credit: NASA/JPL.
I will spare you the 1962 film Journey to the Seventh Planet and move on to new work on Uranus out of the Georgia Institute of Technology, where researchers have discovered that the magnetosphere of the planet ‘flips on and off like a light switch’ (according to this Georgia Tech news release) as it rotates along with the planet. In one orientation, the solar wind flows into the magnetosphere, which later closes to deflect that same wind away from Uranus.
Rotating on its side, Uranus also has a magnetic field that is off-centered and tilted 60 degrees from its axis of rotation. What we wind up with is rapid rotational reorientation, keeping the field strength turbulent. Carol Paty (Georgia Tech), a co-author of the study, puts the matter this way:
“Uranus is a geometric nightmare. The magnetic field tumbles very fast, like a child cartwheeling down a hill head over heels. When the magnetized solar wind meets this tumbling field in the right way, it can reconnect and Uranus’ magnetosphere goes from open to closed to open on a daily basis.”
Compare this with the Earth, where the magnetic field is nearly aligned with the planet’s spin axis, which means that the magnetosphere spins along with the Earth’s rotation. Even so, disruptive events can occur because of geomagnetic storms. A strong solar storm disrupting the magnetic fields of the solar wind can reconfigure Earth’s field from closed to open, rearranging the local magnetic topology to allow a surge of solar energy to enter the system. Such reconnection happens throughout the Solar System, and occurs when the direction of the heliospheric magnetic field is opposite to a planet’s magnetospheric alignment.
Geomagnetic storms produced by coronal mass ejections slamming into Earth’s magnetic field can produce spectacular aurora events while playing havoc with radio communications. Reconnection drives the entire process, happening in the presence of plasma, which carries its own magnetic fields in a constantly adjusting dance between charged particles and fields. Sudden changes in the alignment of the magnetic field lines convert the stored energy of the magnetic fields into heat and kinetic energy that drives particles along the field lines.
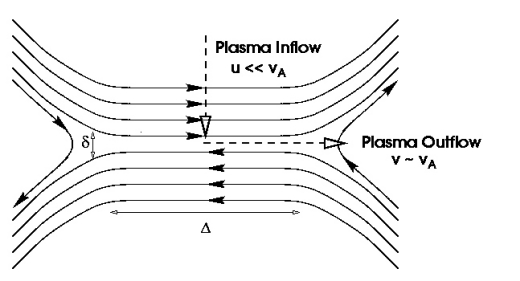
Image: Magnetic reconnection (henceforth called “reconnection”) refers to the breaking and reconnecting of oppositely directed magnetic field lines in a plasma. In the process, magnetic field energy is converted to plasma kinetic and thermal energy. Credit: Magnetic Reconnection Experiment.
Thus a surge of energy enters the system. Such magnetic reconnection should produce auroras at various latitudes on Uranus even if they are a challenge to observe from Earth. The Georgia Tech team’s numerical models predict the most likely places on the planet for reconnection, working with Voyager 2 data from the 1986 flyby. Lead author Xin Cao comments:
“The majority of exoplanets that have been discovered appear to also be ice giants in size. Perhaps what we see on Uranus and Neptune is the norm for planets: very unique magnetospheres and less-aligned magnetic fields. Understanding how these complex magnetospheres shield exoplanets from stellar radiation is of key importance for studying the habitability of these newly discovered worlds.”
The paper is Cao and Paty, “Diurnal and seasonal variability of Uranus’s magnetosphere,” published online by the Journal of Geophysical Research: Space Physics 27 June 2017 (abstract).
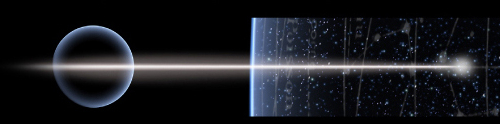
by Paul Gilster | Jun 28, 2017 | Outer Solar System |
When you find a protoplanetary disk that displays unusual properties, the suspicion grows that an unseen planet is causing the phenomenon. The young Beta Pictoris is a classic case in point: Here we see disk asymmetry, with one side of the disk appearing longer and thinner than the other, and a warp that could be caused by the planet known as Beta Pictoris b.
Or consider an extreme case, HD 142527. A T Tauri star in Lupus, HD 142527 displays an inner disk that is tilted by about 70 degrees (see HD 142527: Shadows of a Tilted Disk). Such a striking offset could be caused by an encounter with another star, though there no good candidates. Are we seeing the effects of proto-planets?
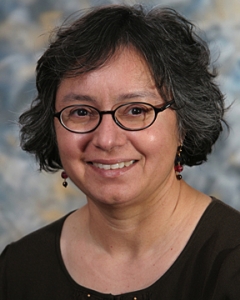
All this comes to mind because of what Kat Volk (JPL) and Renu Malhotra (Lunar and Planetary Laboratory, University of Arizona) are seeing in our own Solar System. Their analysis of the more distant regions of the Kuiper Belt shows that objects there display an offset of about eight degrees from the so-called ‘invariable plane’ of the Solar System.
That tilt needs explanation, and the authors of the new paper speculate that it’s the result of an unseen mass. We may be dealing with a Mars-sized object orbiting roughly 60 AU from the Sun, its orbit tilted by that same eight degrees to the average plane of the known planets.
Image: Lunar & Planetary Laboratory scientist Renu Malhotra. Credit: UA.
Let’s dig a little deeper into this. By the ‘invariable plane’ the authors mean the average inclination of objects orbiting the Sun. In our system, most of this effect comes from the angular momenta of the gas giants, and we find that the plane is within 0.5° of the orbital plane of Jupiter. No surprise there, but when Volk and Malhotra studied the orbital planes of some 600 Kuiper Belt objects, they found the aforementioned tilt of eight degrees in KBOs from 50 to 80 AU from the Sun.
While closer KBOs remain near the invariable plane, more distant KBOs do not. The researchers believe the finding is statistically unlikely to be a fluke due to the the observational sample, putting the possibility in the 1-3% range. Calculating the effects of a planetary mass object on a band of KBOs from 50 to 80 AU, Volk and Malhotra believe they are seeing the effect of something larger than a typical dwarf planet.
Whether or not we are dealing with something as massive as a planet depends upon the strength of alternative explanations. The authors discount the idea that a passing star could have disrupted the Kuiper Belt. From the paper:
… such a perturbation would have to have occurred recently enough that subsequent secular precession of these new orbit planes about the invariable plane has not had sufficient time to relax the KBOs’ mean plane. The precession timescale at a = 50 au is ? 5 Myr and it is ? 15 Myr at a = 80 au. This means that any transient impulse perturbation of the mean plane of objects in this semi-major axis range will tend to be erased by differential secular precession about the invariable plane on timescales of ? 10 Myr. This timescale implies a perturbation much too recent to be a result of stellar flybys (e.g., Levison et al. 2004) or rogue planets (e.g., Gladman & Chan 2006).
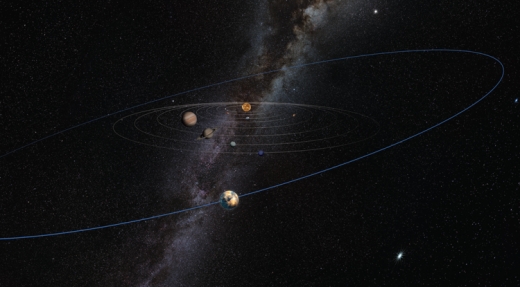
Image: A yet to be discovered, unseen “planetary mass object” makes its existence known by ruffling the orbital plane of distant Kuiper Belt objects, according to research by Kat Volk and Renu Malhotra of the UA’s Lunar and Planetary Laboratory. The object is pictured on a wide orbit far beyond Pluto in this artist’s illustration. Credit: Heather Roper/LPL.
A planet in the outer Solar System inevitably calls up thoughts of the still sought Planet 9 (or, if you prefer, Planet X), a massive object if it exists, and one predicted to be as much as 700 AU from the Sun. Malhotra and Volk are working with something much closer:
Achieving a significant change of the mean plane in the 50–80 au semi-major axis range requires a much closer perturber, because, absent a secular resonance, the forced plane of a KBO is nearly unaffected by an additional planet unless the KBO’s semi-major axis is sufficiently near that of the planet.
We can say a few things about such an object. The authors argue that the observed population of KBOs in the 50 to 100 AU range places an upper limit on its mass. Too much mass and the object would have cleared this region of KBOs over the age of the Solar System — the largest possible value for its mass can be calculated at 2.4 Earth masses. A Mars mass object on a moderately inclined orbit at a semimajor axis of 65-80 AU fits the data well.
As to why such an object has yet to be detected, the authors note the difficulties working in regions near the galactic plane and also point to unsurveyed regions at higher ecliptic latitudes where relatively bright objects (magnitude ~ 17, assuming an albedo based on an icy dwarf planet’s surface) might be hard to spot. “It appears not impossible that a perturber on the order of Mars’ size and mass, at such close distances (? 65–80 au, as required to perturb the Kuiper belt’s mean plane) remains to be discovered.”
This University of Arizona news release offers Malhotra’s speculation that the Large Synoptic Survey Telescope, scheduled for first light in 2020, may be able to spot the object. Indeed, the instrument’s surveys are expected to increase the number of observed KBOs from 2000 to 40,000, an increase great enough to offer hope that we’ll find any objects like these.
The paper is Volk & Malhotra, “The curiously warped mean plane of the Kuiper belt,” accepted at the Astronomical Journal (preprint).
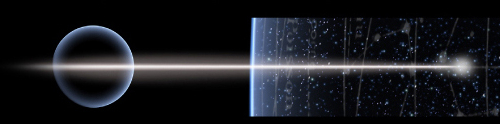
by Paul Gilster | Jun 27, 2017 | Outer Solar System |
Our spacecraft have never encountered an object as far from Earth as 2014 MU69, but New Horizons will change all that when it races past the Kuiper Belt object on New Year’s Day of 2019. This summer is an interesting part of the project because planners will use it to gather as much information as possible about what they’ll find at the target. We have three occultations to work with, one of them just past, and they are as tricky as it gets.
But before I get to the occultations, let me offer condolences to the family and many New Horizons friends of Lisa Hardaway, who died in January at age 50. Hardaway helped to develop the LEISA (Linear Etalon Imaging Spectral Array) spectrometer that brought us such spectacular results during the Pluto/Charon flyby. She was program manager at Ball Aerospace for the Ralph instrument that contains LEISA. Mission scientists used data from the instrument package to make geological, color and composition maps of Pluto and its moons. The mission team has now dedicated the spectrometer in her memory.
“Lisa played a critical role on several of Ball’s more significant scientific programs from New Horizons, Hubble and Orion, to the Boeing F/A 18 E/F fighter jet and other national defense projects,” said Rob Strain, president of Ball Aerospace in Boulder, Colorado. “She made a positive impact on the careers of many, and her contributions will continue to give back for years to come.”
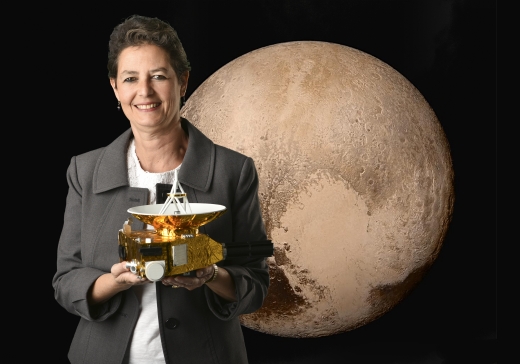
Image: Aerospace engineer Lisa Hardaway with the New Horizons spacecraft and Pluto. Hardaway was program manager for the Ralph instrument, which captured the first close-up color images of Pluto. Credit: Ball Aerospace.
Tracking a Fleeting Shadow
The MU69 occultations should provide useful data as we learn more about the KBO encounter environment. The first event occurred on June 2-3, with observers in Argentina and South Africa — 54 telescope teams in all — trying to track the shadow of MU69, which had occulted a star. Alan Stern, principal investigator for New Horizons, explains the goal:
“A tremendous amount had to go right to correctly execute such a massive observation campaign, but it did. The main goal of these observations was to search for hazards; the secondary objective was to try to glimpse the occultation of MU69 itself, in order to learn its precise size. Scouring all the dozens of datasets for these two objectives is going to take us a few weeks.”
Predicting the narrow stripe of MU69’s shadow over the Earth called for data from the European Space Agency’s Gaia mission as well as the Hubble Space Telescope. Remember that it was only in 2014, after a determined search, that the Hubble instrument discovered the 45-kilometer object, which is only 1/10,000th the mass of Pluto, although ten times larger than the average comet. Just the kind of KBO we’d like to study, in other words, but one we’ve had to characterize quickly in preparation for the upcoming flyby.
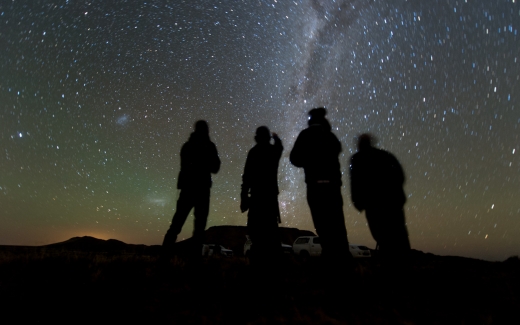
Image: Four members of the South African observation team scan the sky while waiting for the start of the 2014 MU69 occultation, early on the morning of June 3, 2017. The target field is in the Milky Way, seen here from their observation site in the Karoo desert near Vosburg, South Africa. They used portable telescopes to observe the event, as MU69, a small Kuiper Belt object and the next flyby target of NASA’s New Horizons spacecraft, passed in front of a distant star. Credits: NASA/JHUAPL/SwRI/Henry Throop.
As the data from the recent occultation are analyzed, we can look forward to another on July 10 and a third on July 17. For the July 10 event, mission scientists will use the Stratospheric Observatory for Infrared Astronomy (SOFIA), a 2.5-meter airborne telescope mounted in a Boeing 747SP jet. SOFIA can work at 45,000 feet, well above intervening clouds and capable of providing better data than the army of small telescopes used in the June occultation.
For the July 17 occultation, two dozen 40-centimeter telescopes will be deployed to Patagonia, where observers hope to scan more deeply for any debris around MU69. The star being occulted will be the brightest of the three, offering the best prospect for such detections. To track all this, keep an eye on the New Horizons KBO Chasers page, but as we get close to the event check the project’s Facebook page and the Twitter hashtag #mu69occ.
As of this morning, we’re 689,472,210 km from MU69 with 553 days to go until flyby.
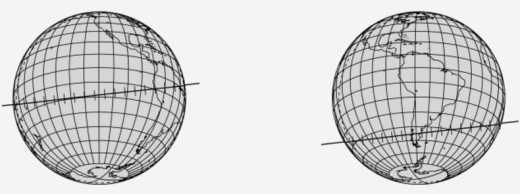
Image: Projected path of the 2014 MU69 occultation shadow, on July 10 (left) and July 17, 2017 (right). Credits: Larry Wasserman/Lowell Observatory.
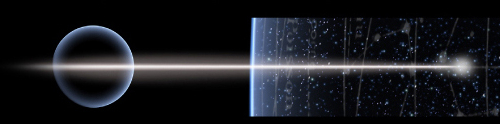
by Paul Gilster | Jun 23, 2017 | Sail Concepts |
Breakthrough Starshot, the ambitious 30-year plan for launching small interstellar craft to a nearby star, depends critically on the sails that will ride a laser beam to 20 percent of lightspeed. In the essay below, James Benford takes a hard look at where we are now in the matter of sail stability, a subject he and brother Gregory have analyzed in their laboratory work. But as Jim points out, there is a great deal we still don’t know, emphasizing the need for a dedicated test facility in which deep analysis and experimentation can proceed. The Chairman of the Sail Subcommittee for Breakthrough Starshot, Dr. Benford gives us insight into the magnitude of the challenge, and the possible solutions now being considered.
By James Benford
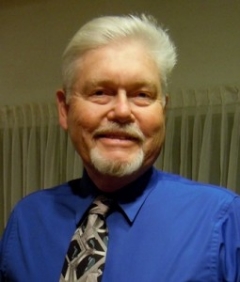
Riding on the beam, i.e., stable flight of a sail propelled by beam momentum, is an essential requirement of beam-driven propulsion. It places considerable demand upon the shape of the sail and beam. Some amount of beam jitter, oscillations in the location of the beam, is to be expected. But even if the beam is steady, a sail can wander off the beam and its shape can become deformed by the high acceleration. Little of the parameter space of possible sail shapes and materials, as well as beam shapes, has been explored to date. Here I describe recent developments.
Sail Geometries
Generally, sails without structural elements cannot be flown if they are convex toward the beam, as the beam pressure would act to compress and perhaps collapse them. On the other hand, beam pressure keeps concave shapes in tension, so conical concave shapes are a possible natural answer to sailship configuration questions, essentially a circular cone. These shapes resist sideways motion if the beam moves off-center, since a net sideways force restores the sail to its original position.
Simulations show that this passive stability works, but it requires suspending the payload below the sail for a more stable configuration. This configuration is a conical shape, perhaps spinning, with the payload hanging from the apex along a tether, which may be flexible, as in Figure 1. It is shaped roughly like a parachute. (Although sails have stress distributions different from a parachute: The beam pressure keeps concave shapes of sail under tensile stress, but the periphery of the sail tends to close, so the sail needs a ring at the largest radius to keep it unfurled. This ring is under compression, and subject to elastic instability.) Such a ring may be a logical place to put the payload, which I think should be distributed to avoid single point failures. I’d much rather have it around the periphery and with some redundancy. One thing I’d don’t like about the “chipsail” idea is that having a single few square centimeter chip on a few square meter sail means that one unfortunate interstellar dust impact will obliterate the payload, losing the mission.
Simulations of conical sails showed the boundaries of sail shape and payload mass giving stability [1]. Simulations at JPL and University of New Mexico showed stability for sail shapes with the conical surface at angles from the horizontal of 25 to 30 degrees, a narrow range. Experiments have verified that beam-riding restoring forces do occur [2]. Stability also depends upon the ratio of the sail mass to payload mass. A recent analysis by Popova et al. showed analytically that stability occurs when the distance from the sail to the center of mass of the entire assembly is larger than the sail radius of curvature for small radius of curvature-L greater than D, the sail diameter [3]. These results mean that the tether must be several times the sail diameter. The mechanical oscillation modes of the tether/payload/sail that affect stability will require carefully analysis.
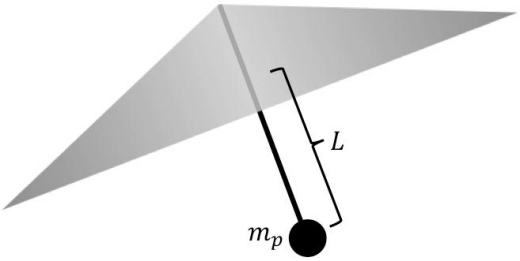
Figure 1 Conical sail with payload of mass mp hanging beneath it, connected by a tether or pendulum bob of length L (Credit: Z. Manchester).
Manchester & Loeb have suggested a spherical sail-hollow, like a shiny balloon, riding on a hollowed-out ‘donut’ beam shape [4]. This architecture has passive stability while also allowing the payload to be inside the sphere, shielded from the laser beam. That’s because it produces a potential well for the sail to sit in, which is stable if the well is deep enough. But the well in the figure is rather shallow. The sail can be inflated into the spherical shape, so could be gas-filled. This hollow beam, composed of 4 Gaussians is just one of many possible “hollow beam” geometries.
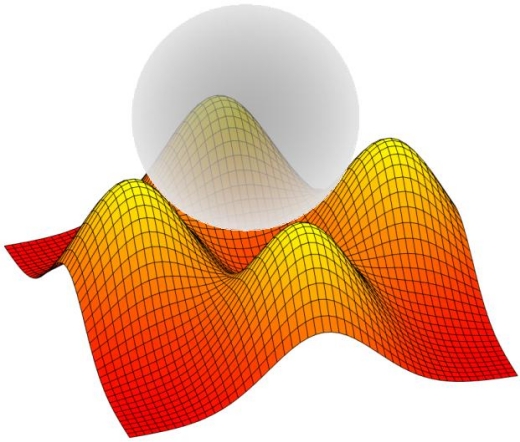
Figure 2 Multimodal beam profile composed of four Gaussian laser beams with spherical sail immersed in them. The corresponding ‘potential well’ for the transverse dynamics of a sail is much shallower than this beam intensity profile. (Credit: Z. Manchester).
From [4] I calculate that, in the example profile of figure 2, 80% of the beam power is lost to the sides of the sail in order to produce this stable regime. That would require that the power of the laser be increased to factor of 5, a huge cost which may be improved using a ring beam with no low areas, through which the sail can escape. Therefore the cost of the spherical sail-hollow beam approach is a big drawback.
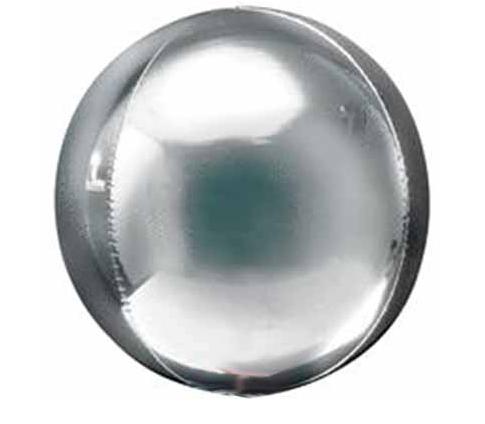
Figure 3 A spherical sail will resemble this helium-filled aluminized Mylar balloon. The beam pressure can cause the beam-facing side of the sail to flatten, assuming a more hemisphere shape.
The spherical sail is an idealization akin to that of the “spherical cow” of theoretical physics infamy. In practice, material under about 10,000 g’s acceleration will not remain spherical. It will be deformed under acceleration into an oblate shape, and therefore the spherical symmetry will cease. So will the stability guarantee. The beam pressure causes the beam-facing side of the sail to flatten, so it assumes a more hemisphere shape. Such deformation of the sphere’s surface could cause significant torques on the sail, complicating analysis. This should be explored by modeling and numerical simulations.
Finally, a spherical sail maximizes cross-section to interstellar dust & gas. Compare to a shallow cone, rotated to go edge-on to the direction of travel. The spherical sail must undergo topology change to reveal the payload, and then reconfigure to become an antenna for return of data.
Modeling Sail Flight
Models of sail stability have thus far assumed a perfectly rigid sail. To deal with the many factors involved in sail stability, simulations must relax the rigid body constraint of work up to now. An example of a code geometry that has been studied in simulation is shown in Figure 4.
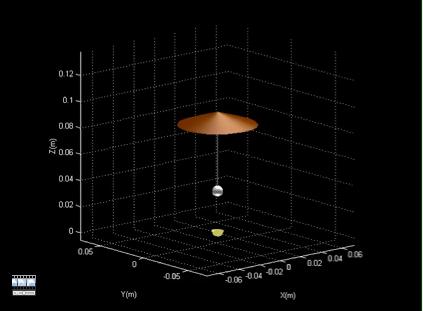
Figure 4 Simulation space for a rigid conical sail with pendulum bob payload [1]. The beamer is directly below the sail.
To address the beam-riding challenge, future simulation codes should include
- a large variety of sail shapes and beam profiles,
- multiple internal reflections,
- sail vibration modes,
- sail spin,
- simulations must include sail material characteristics including reflection/absorption as a function of angle of incidence.
Codes must also model noise in the system, arising from factors such as perturbations due to atmospheric turbulence, laser pattern variations as well as jitter in the beam and vibrational modes of the sail. While perturbations of the sail will surely excite oscillatory motion, the sail must be in a sufficiently deep ‘potential well’ to remain on the beam.
Conclusion
The parameter space of sail shape and beam shape has been studied to only a narrow, easily accessible extent. The conical sail with a payload hanging below at is the most studied and appears to have some stable regimes. But this is verified only by analytic modeling and limited simulation of the equations of motion. Mechanical aspects of the tether/payload/sail will affect stability and has not been explored. The spherical sail has been studied analytically and appears to be stable only inside a hollow beam, which substantially reduces the efficiency of propulsion.
There is much theoretical and experimental work to be done on beam-riding, an essential requirement of beam-driven propulsion. Beam-riding and sail stability analytical models have reached their limits. Computational models must be substantially improved. Such models can be validated by laboratory experiments. For such a complex problem, only experiments will be decisive.
Looking beyond the distant goal of Star Shot, beam powered sails can play a role in the coming era of interplanetary exploration and eventual commerce. The first useful beam-riding sails will come from such stability studies, which should be undertaken now. In this lower velocity and power regime the dynamics will play out. Such lessons can have great use over decades to come.
[1] D. Georgiev, E. Schamiloglu, C.T. Abdallah and E. Chahine, “3-D Simulations of Rigid Microwave Propelled Sails Using Spin”, Beamed Energy Propulsion, AIP Conf.
Proc. 664, pg. 336, A. Pakhomov, ed., 2003.
[2] G. Benford, O. Goronostavea and J. Benford, “Experimental Tests Of Beam-Riding Sail Dynamics”, Beamed Energy Propulsion, AIP Conf. Proc. 664, pp. 325, A.
Pakhomov, ed., 2003.
[3] H. Popov, M. Efendiev and I. Gabitov, “On the stability of a space vehicle riding on an intense laser beam”, Mathematical Methods in the Applied Sciences, John Wiley & Sons, Inc., 40, pp. 1346, 2016.
[4] Z. Manchester and A Loeb, “Stability of a Light Sail Riding on a Laser Beam”, ApJL 837 L20, 2017.
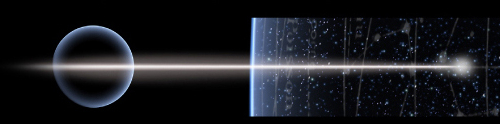